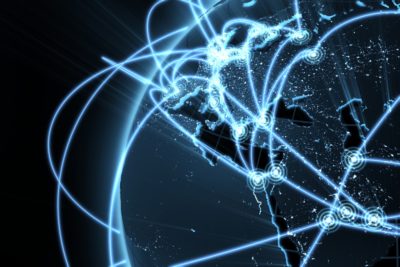
“Our prime purpose in this life is to help others. And if you can’t help them, at least don’t hurt them.”
– 14th Dalai Lama
Neurobiology of Pain – An Introduction
Pain
Pain is defined as “an unpleasant sensory and emotional experience associated with actual or potential tissue damage, or described in terms of such damage.”
Because the preferred choice of treatment for pain is based at least in part on the type of pain, it is important to understand the different types of pain and how to distinguish one type from the other. The experience of pain is often a combination of different types and therefore the treatment of pain often benefits from the use of more than one approach and/or type of pain medication/treatment.
See also:
Gabapentin (Neurontin) & Pregabalin (Lyrica)
Toll-Like Receptor Antagonists (TLR-4)
Definitions and Terms Related to Pain
Key to Links:
Grey text – handout
Red text – another page on this website
Blue text – Journal publication
.
Neurobiology of Pain
Pain Terms:
Nociception
Nociception refers to the sensory nervous system’s detection and response to harmful or potentially harmful stimuli. In nociception, intense chemical, mechanical, or thermal stimulation of sensory nerve cells called nociceptors produces signals that travel from the source along a chain of nerve fibers to the spinal cord and then through “ascending pathways” from the spinal cord to the brain.
Nociceptive Pain
Nociceptive pain is caused by activation of neural pathways in response to damaging or potentially damaging stimuli to body tissue such as occurs with chemical or mechanical trauma or burns. It is usually described as a sharp, aching, or throbbing pain. Nociceptive pain is further divided into “visceral” pain, originating from the organs inside the body, and “somatic” pain, originating from muscles, bone and skin. Visceral pain is often vague, difficult to describe and hard to localize. Nocicptive pain is usually a symptom of a disease process.
Nociception is the sensory nervous system’s response to harmful or potentially harmful stimuli. In nociception, intense chemical, mechanical, or thermal stimulation of sensory nerve cells called nociceptors produces signals that travel from the source along a chain of nerve fibers to the spinal cord and then through “ascending pathways” from the spinal cord to the brain.
“Neuropathic” or nerve pain is pain initiated or caused by a primary lesion or dysfunction in a nerve or in the nervous system or pain arising as a direct consequence of a lesion or disease affecting the nervous system. Nerve pain is usually perceived as burning, electric, shock-like, tingling or sharp and may start at one location and shoot, or “radiate” to another location (like sciatica). Neuropathic pain can be “peripheral,” (outside the central nervous system),” like carpal tunnel pain or “central,” originating in the spinal cord or brain. Neuropathic pain is often a disease process, not simply the symptom of one.
For more information: Assessment and Management of Neuropathic Pain
The Distinction between Nociceptive and Neuropathic Pain
Neuroscientists use distinct pain models for nocicptive (non-neuropathic) pain and neuropathic pain because of different proposed mechanisms, different experiential perceptions and, most importantly, to guide effective treatment options. In many ways making this distinction offers advantages to the management of chronic pain. That being said, the actual distinction between these two categories of pain are blurred.
The same neurotransmitters, neuropeptides, cytokines, and enzymes are implicated in both types of pain, with a large degree of overlap. Opioids are effective for both types of pain, though less so for neuropathic pain often requiring higher doses than for nociceptive pain. NMDA receptor antagonists (ketamine, and others) are often considered to be effective for neuropathic pain only, being intricately involved in the process of central sensitization, but preclinical and clinical studies have shown that they reduce nociceptive pain also.
It is important to note also that ascending spinal pathways, supraspinal regions that process these signals, and descending modulation pathways are essentially the same for neuropathic and nociceptive pain. All of the ways that pain signalling can be modified at any of these areas will affect both neuropathic and nociceptive pain. The rest of the neuromatrix of the pain experience, including brain reorganization and emotional interplay are also shared between both neuropathic and nociceptive pain.
In conclusion, although treatment based on the mechanism(s) of pain is widely accepted to be theoretically better than treatment based on the cause of pain, or empirical treatment, this paradigm of neuropathic vs. nociceptive pain should encourage flexibility in pain management as implemented in clinical practice.
Hyperalgesia
Hyperalgesia is an exaggerated, increased painful response to a stimulus which is normally painful and is classified as either primary or secondary. Primary hyperalgesia accompanies injury to tissue as an outcome of peripheral nociceptor sensitization. In contrast, secondary hyperalgesia is found in the neighboring intact tissue because of the sensitization of the central nervous system. It is thought that this sensation can be attributed to either the extension of the receptive fields of damaged nerves, or by transmission of excitatory signals that spread through neighboring uninjured nerve fibers.
see also: Assessment and Management of Opioid Induced Hyperalgesia
Allodynia
Allodynia is a term that descri
bes the experience of pain arising from a stimulus which does not normally provoke pain. This pain can stem from undamaged neighboring fibers, the dorsal root ganglion in the spinal cord, or along other points of the damaged nerve. Allodynia may result from reduced nerve thresholds and is usuallly characterized as either thermal or mechanical in nature. Allodynia is a common symptom associated with fibromyalgia and migraine headaches but may arise as a result of other chronic painful conditions possibly related to central sensitization.
For more information: Assessment and Management of Central Sensitization
Central Sensitization is a process of hyper-responsiveness to sensory stimuli which is a result of chronic pain-induced changes in the spinal cord and brain. It can be an important contributing process to the chronic pain experience.
For more information: Assessment and Management of Central Sensitization
Peripheral Sensitization
Sensitization and stimulation of nociceptors, both of which can be caused by numerous factors, effectively lower neuronal thresholds of action potentials and promote peripheral sensitization. These factors include inflammatory mediators that are discharged from nociceptive terminals, including substance P and the calcitonin gene-related peptide. These mediators promote vascular permeability, which causes local edema and the leakage of growth factors, prostaglandins, cytokines, and bradykinin. It is thought that the multifactorial dependence of various substances on producing nociceptive sensitization may explain why there is no single comprehensively effective drug.
Anatomic Terms
Receptors and Neurotransmitters
Receptors are cellular structures, usually a complex protein molecule found on the surface of a cell, that interacts or binds with a chemical which then triggers a response. These chemicals may be neurotransmitters (in the nervous system), hormones or drugs. A chemical or drug that triggers a reaction with a receptor is referred to as an “agonist,” while one that blocks the activity of a receptor is referred to as an “antagonist.”
Opioid Receptors
In the world of pain, there are a handful of well-studied receptors that play prominent roles, most notably the opioid receptors which interact with both endogenous opioids (opioids manufactured by the body) and exogenous opioids (opioid medications). Opioid receptors are distributed widely in the brain and spinal cord, particularly in areas of the nervous system associated with pain perception and transmission. Opioid receptors are also found in the digestive tract where they play a key role in how opioid medications can cause constipation.
NMDA Receptors
Besides the opioid receptors, another receptor that plays a significant roles in pain, especially nerve pain, is the NMDA receptor. It remains uncertain how NMDA and opioid receptors interact but blocking the NMDA receptor appears to reduce the development of opioid tolerance and opioid induced hyperalgesia. The list of receptors important in pain is not limited to only these few receptors but these are the receptors that when understood can provide insights towards better choices for treating pain. Thus, understanding the nature of a person’s pain and understanding the difference in how medications work allows for tailoring an individualized pain management plan to optimize pain control. (For more information about opioid and NMDA receptors, see Neurobiology of Opioids).
Pain Perception and the Pain Experience
An Overview of Pain Processing
Signals from pain receptors in the peripheral nervous system (nociceptors – types A and C), send signals to the central nervous system, starting in the dorsal horn of the spinal cord. From dorsal horns, pain signals travel to the brain, (thalamus and midbrain) via the ascending spinal pathways including the spinothalamic tract. The thalamus acts as a relay station for processing the pain information. Pain signals are then transmitted to somatosensory cortex to localize and characterize the pain, as well as other areas in the cortex (ee below).
Corresponding projections to limbic regions of the brain are involved in processing elements of pain including emotion and memory. Pain signals are processed both consciously and unconsciously. The final experience of pain reflects the input and modification of pain signals from the pain source from these different areas of the brain, reflecting a person’s experiences as affected by their emotions and memories.
The brain sends signals to descending pathways to modulate (change or inhibit) pain impulses. These descending fibers release substances (endogenous opioids, serotonin, and norepinephrine) that bind to the opioid receptors and prevent the release of the neurotransmitters such as glutamate or Substance P (SP), thereby blocking the pain signal from being transmitted.
Phasic vs Tonic Pain
There are two types of pain: phasic and tonic. Phasic pain is acute, brief, rapidly rising, transient, and well-localized. Injury-produced persistent, chronic and inescapable pain is tonic pain. The suppression of phasic pain is mostly controlled through the brainstem and descending pain-suppression neural pathways. The inhibition of tonic pain, however, requires the activation of neural systems in addition to those required to inhibit phasic pain. The systems involved in the suppression of tonic pain included forebrain sites, rostral to the brainstem, including the dopamine neurons in the reward area of the brain.
Brief Overview of the Pain Circuits
The first step in perceiving pain is the result of stimulation of “nociceptors,” special pain receptors located in the periphery of the body including skin, bone and other tissues . The nociceptors send signals to an area of the spinal cord called the dorsal horn. In turn, signals are than sent from the dorsal horn to the brain via neural circuits called the “ascending pathways” (See below for more about the ascending pathways). When the brain receives these signals, additional processing occurs in higher brain centers allowing conscious awareness and emotional response to the painful stimulus.
Nociceptors
Nociceptors are specialized sensory nerves that are responsive to damaging or potentially damaging stimuli including temperature, mechanical, chemical stimuli as well as others that have variable functions. They are found in any area of the body that can sense noxious stimuli either externally or internally. The cell bodies of these nociceptors neurons are located in either the dorsal root ganglia (DRG) in the spinal cord or the trigeminal ganglia that serve the face and head. The DRG is a site in the spinal cord where pain signalling is modified by other nerves and a site where naturally occuring opioids, the enkephalins and endomorphins, act to influence pain perception. The DRG is also thought to be a contributing site for the evolution of central sensitization.
Nociceptors send signals to the spinal cord and, via the ascending spinal pathways, to the brain in a process called “nociception” resulting in the conscious perception of pain. The nociceptors are a site of action for topical pain medications including capsaicin, ketamine, anesthetics and other drugs.
Ascending Spinal Pathways
Signals from nociceptors transmitted to the dorsal horns in the spinal cord are transmitted via secondary order neurons to the brain via ascending spinal pathways. One of these pathways, the spinothalamic tract, carries pain signals to the thalamus and midbrain. This area of the brain acts as a relay station for processing the pain information including interactive nerve pathways, or circuits, to other parts of the brain including higher cortical centers.
Pain processing in the brain
www.neuroscientificallychallenged.com/know-your-brain
It is important to emphasize that these brain regions are not selectively or exclusively activated by nociception or restricted solely to pain perception. For example, the mesolimbic reward system is activated by natural rewards and rewarding drugs. Regions serving different neurological functions including cognition, emotion, motivation and sensation are functionally connected in the context of nociception and together give rise to the experience of pain. The interactions among these sites also provide a means whereby emotional and motivational cues can alter the experience and perception of pain.
Cortical Centers:
The anterior cingulate cortex:
Involved in anxiety, anticipation of pain, attention to pain, and motor responses
The primary and secondary somatosensory cortices (S1 and S2) and the insular cortex:
The primary and secondary somatosensory cortices localize and interpret noxious stimuli. The insular cortex may play a role in the sensory discriminative and affective aspects of pain that contribute to the negative emotional responses and behaviors associated with painful stimuli. The somatosensory cortices (S1 and S2) and the insular cortex are believed to encode the sensory features of pain, which include quality (stinging, burning or aching), location and duration.
Mesolimbic System
The mesolimbic system, or pathway connects the ventral tegmental area in the midbrain, to the ventral striatum of the basal ganglia in the forebrain. The ventral striatum includes the nucleus accumbens (NAc) and the olfactory tubercle. The mesolimbic pathway, referred to as the reward pathway, is a dopaminergic pathway. Alterations or dysregulation of the mesolimbic pathway and the nucleus accumbens play a significant role in the pathology of chronic pain and in the development and maintenance of addiction.
The prefrontal cortex:
The prefrontal region and the limbic system (ACC, Prefrpmta; Cortex (PFC), amygdala, Ventral Tegmental Area (VTA) and Nucleus Accumbens (NAc) encode emotional and motivational responses, and are implicated in the affective and contextual aspect of pain. The prefrontal cortex is important for sensory integration, decision making, memory retrieval, and attention processing in relation to pain.
Descending pain pathways from the cortices to the mesolimbic areas
Mesolimbic pain-related areas are modulated by higher cortical areas, such as the prefrontal cortex, through a descending pain control system. While the neurotransmitter most commonly associated with descending pain modulation is opioid, others such as dopamine and cannabinoids also play a role.
Midbrain
Periaquaductal Gray (PAG)
The midbrain PAG is the primary control system for descending modulation of nociception as well as a host of other autonomic and emotional behaviors.
The Nucleus Accumbens (NAc):
The nucleus accumbens is in an area of the brain called the basal forebrain, one in each hemisphere, situated between the caudate and putamen. The nucleus accumbens is part of the basal ganglia and is also the main component of the ventral striatum. The nucleus accumbens itself is separated into two anatomical components: the shell and the core.
The NAc regulates incentive salience (i.e., motivation and desire for rewarding stimuli) and facilitates reinforcement and reward-related learning. It also plays a role in the perception of pleasure and the sense of well-being and is involved in placebo analgesia. The NAc plays a key role in motivating appetitive behaviors (i.e. natural rewards such as drinking, eating and reproduction); Neural pathways involving there NAc are engaged by drugs that are intrinsically rewarding and can lead to addiction.
The amygdala, hippocampus, and other parts of the limbic system:
Which are involved in the formation and storage of memories associated with emotional events, aect, arousal, and attention to pain and learning. The limbic system may also be partially responsible for the fear that accompanies pain.
The Neuromatrix
The final experience of pain is a result of modifications by various centers in the brain that communicate together, by emotion and personal experience, by genetics – all of which are referred to as the “Neuromatrix,” which make their final impact on the higher, cortical areas of the brain that reflect the actual conscious experience of pain.
The neuromatrix theory of pain provides a new conceptual framework to understand pain. It proposes “that the output patterns of the body-self neuromatrix activate perceptual, homeostatic, and behavioral programs after injury, pathology, or chronic stress. Pain, then, is produced by the output of a widely distributed neural network in the brain rather than limited to sensory input evoked by injury, inflammation, or other pathology. The neuromatrix, which is genetically determined and modified by sensory experience, is the primary mechanism that generates the neural pattern that produces pain.” The final pain pattern is determined by multiple influences, of which the body’s sensory input is only a part, that converge on the neuromatrix.
Thus, the pain experience is a function of signals triggered by peripheral receptors that travel to the spinal cord, then to the brain and then back to the spinal cord. The experience of pain can be modified by emotional reactions and other brain processing that affects the function of any of these components in the pain processing system as well as by medications interacting on any of these components.
Descending Pathways
After processing pain signals, the brain then sends signals back to the dorsal horn of the spinal cord via the “descending pathways.” which for the most part are inhibitory, meaning they act to diminish the perceived severity of the pain experience (See below for more about the descending pathways).
The descending pathways send signals from the brain to the spinal cord that inhibit pain (dorsal horn and DRG of the spinal cord via the rostral ventromedial medulla and the periaqueductal grey (PAG) of the midbrain). In the dorsal horn of the spinal cord, serotonin, noradrenaline (norepinephrine) and other neurotransmitters are released then bind to nerves in the spinal cord. These nerves regulate the influx and transmission of pain signals, allowing for increasing or, usually, decreasing pain signals. As a neurotransmitter, serotonin sometimes increases pain and sometimes inhibits pain, depending on the nerve pathway. Noradrenaline is mostly inhibitory to pain. The antidepressant medications such as Cymbalta, Effexor and Savella as well as some opioids (tramadol, tapentadol, buprenorphine and levorphanol) work on these pathway by inhibiting the reuptake of serotonin and noradrenaline that results in enhancing their inhibition of pain. It appears that it is the noradrenaline that plays the greatest role in inhibiting pain, especially nerve pain.
The brainstem descending pain-suppression system, however, plays a more important role in the suppression of brief, rapidly rising, transient, and well-localized (i.e., phasic) pain than it does in the suppression of injury-produced persistent (i.e., tonic) and inescapable pain. However, several lines of evidence suggest that the inhibition of the tonic pain requires the activation of neural systems in addition to those required to inhibit phasic pain.
Opioids can inhibit pain by acting at spinal sites and at sites in the brainstem, where they modulate activity in descending brainstem pathways projecting to the spinal cord. A primary site of action is the periaqueductal gray of the brainstem where stimulation of opioid receptors activates, through direct projections, serotonin-containing cells in the nucleus raphe magnus. In turn, the latter cells activate neurons that project, via the dorsolateral funiculus, to the dorsal horns of the spinal cord where they inhibit cells that transmit information about noxious painful stimulation from the periphery to supraspinal sites.
Since painful stimuli that are processed by the brain can result in either suppression or increased pain, we look to modify these pathways as one means of treating pain. As noted above, some medications work on these pathways. Sometimes supplements can also affect these pathways, including those that inhibit COMT, an enzyme that metabolizes noradrenaline for example (see Nutrition &
Supplements). It is believed that the pain benefits obtained with mindful exercises such as meditation, prayer, hypnosis, deep relaxation exercises etc. are brought about by increasing these inhibitory signals via the descending pathways. In some cases however, the brain prcessing may result in increased severity of pain such as what may occur when an emotional reaction to pain makes the pain feel worse.
NSAIDs, Opioids, Cannabinoids and the Descending Pain Control System
In the descending pain control system the nerve impulses flow from the forebrain to the spinal cord and other structures to regulate the transmission of reflex and ascending messages by increasing or decreasing sensitivity to pain. Whether the descending pain control system increases or decreases the transmission of pain messages depends on various circumstances.
The periaqueductal gray matter (PAG), located around the aqueduct of Sylvius in the midbrain is a very important structure of the descending pain control system. The PAG gathers information from multiple structures —the somatosensory and cingulate cortices, the amygdala, the thalamus and the hypothalamus—as well as from ascending pain pathways. Different regions of the PAG are involved in different functions.
Regarding descending control of pain, the dorsal-dorsolateral portions of the PAG are involved in stress-induced analgesia, which is independent of opioids but depends on endocannabinoids. The lateral-ventrolateral portions of the PAG are involved in analgesia induced by opioids and NSAIDs. The PAG sends impulses to the nucleus raphe magnus and neighboring structures of the rostral ventromedial medulla (RVM). In the RVM there are two classes of neuron that project to the spinal cord: the on-cells, which facilitate, and the off-cells, which inhibit transmission of pain signals.
Both the PAG and the RVM have many opioid receptors and are greatly involved in the analgesic from both endogenous and exogenous opiates. Opioids activates PAG output neurons to decrease in the activity of RVM on-cells and an increase in the activity of off-cells, to simultaneously reduce facilitation and increase inhibition of spinal nociceptive neuron to reduce pain. Opioids activate the RVM with similar effects. In summary, opiates reduce pain because, in addition to their direct action upon peripheral tissues and the spinal dorsal horn, they activate descending inhibition of pain messages by the descending pain control system.
These mechanisms for analgesia by the descending pain control system are shared by endogenous opioids, NSAIDs and also endocannabinoids. This is consistent with current studies demonstrating additive and synergistic benefits for the treatment of pain with the combined use of opioids with NSAIDs and cannabinoids such as CBD, BCP and THC.
Dopamine and Pain
Opioids stimulate the release of dopamine from these neurons through their indirect activation, whereas psychostimulant drugs such as amphetamine and cocaine increase dopamine extracellularly by decreasing reuptake and/or inducing release. Opioids and psychostimulants both have rewarding and analgesic effects, suggesting that these effects might share a common neural substrates basis. There is evidence that mesolimbic dopamine neurons play a role in the suppression of tonic, but not in the phasic pain. Research also indicates that dopamine agonists that activate D2 receptors in the nucleus accumbens inhibit inflammatory pain. The number and availability of these D2 receptors which play a role in both reward and. pain are influenced by genetic, stress, mood and emotional factors.
In conclusion, this intimate relationship with pain neuro-circuitry and the dopamine-based reward system neuro-circuitry lends credibility to the potential benefit of maintaining dopamine homeostasis (balance) in the management of chronic pain.
Glia Cells and the Mesolimbic Dopamine System
The specifics of how chronic pain affects the mesolimbic dopamine (DA) system is not fully understood, but there is new evidence for a role of altered DA signaling, not only in the chronicity of pain, but also in its response to opioid drugs. This evidence suggests a mechanism in which chronic pain activates microglia in the VTA to inhibit DA transmission and disrupt reward behaviors by disrupting Cl homeostasis in GABAergic neurons. Inhibiting microglial activation restores normal DA signaling and reward behavior. Microglial activation is a critical component modulating reward behavior in chronic pain. Inhibiting microglial activation may be an effective strategy for restoring disrupted DA transmission in Reward Deficiency Syndrome (RDS) as well as disorders linked to RDS such as depression and addiction. Microglial inhibitors such as minocycline, palmitoylethanolamide (PEA) and others are being explored and demonstrating promise as effective agents in chronic pain.
See:
Emotion and Pain
Pain is modulated by various factors, the most notable of which is emotions, including love. Emotions enhance or reduce pain. Recent advances in neuroimaging have revealed similarities in brain activation relating to emotions and pain. At the simplest level, these similarities can be explained by the overlapping network structure in brain functional connectivity, although the explanation is considerably more complex. The determination of whether love can result in increased or decreased pain perception depends on the psychological construct of the individual, as well as the individual’s ability to engage resources relating to emotion regulation. In turn, this determines how a person relates to love and reacts to pain.
The pain of heartbreak activates similar areas of the brain compared to physical pain. The pain of heartbreak also can last longer than that of physical pain, with recollections of the hurt caused by a breakup with a loved one being more vivid than those of previously experienced physical pain. The feelings of pleasure associated with being ‘in love’ have been shown to suppress pain. This love-induced analgesia is associated with activation of the brain areas that code reward, i.e. the caudate nucleus, nucleus accumbens, orbitofrontal cortex, amygdala and dorsolateral prefrontal cortex.
Emotion regulation refers to the ‘processes by which individuals influence which emotions they have, when they have them, and how they experience and express these emotions’, in other words, the alteration of emotional processes for coping purposes. Effective emotion regulation involves skills relating to awareness appraisal, regulation and the adaptive use of emotions. The most commonly implicated brain areas involved in emotion regulation are the areas associated with the reward pathways. The effective use of emotion regulation as a means to control pain has been demonstrated using strategies such as attentional manipulation and reappraisal.
The role of the brain’s opioid system in relieving emotional pain, including social separation and distress, has been identified. It has also been found that social acceptance positively correlates with activation of the μ-opioid receptors in the reward pathways including the nucleus accumbens. The opioid system plays a role in modulating feelings of love and rejection. However, this effect is absent in patients with major depressive disorder.
This neural similarity within the pain and love networks may explain the manifestation of social pain and the modulation of pain by emotion. Interestingly, it has been demonstrated
in one study that the painkiller acetaminophen, used to treat physical pain, is also effective in reducing emotional pain. Intense, passionate romantic love has been referred to as a natural addiction, with similar manifestations to substance, non-substance and behavioral addiction, including euphoria, craving, tolerance, emotional and physical dependence, withdrawal and relapse. Powerful emotions also involve the dopamine brain reward center as do addictions. It also suggests a basis for the use of opioids in the addictive process for coping the emotional pain of reward deficiency.
The interaction between the ‘love’ and ‘pain’ networks suggests possibilities for management of pain. Oxytocin, the ‘love hormone,’ and vasopressin, are the most prominent hormones implicated in pair bonding. Oxytocin when administered as a drug has demonstrated potential to suppress pain.
Stress and Pain
High levels of stress lead to chronic states of fatigue and depression as well as an amplification of chronic pain. Indeed, an individual with chronic pain is definitely in a stressful condition leading to a vicious cycle of pain amplifying stress amplifying pain. Management of chronic pain is inextricable from the need to manage stress.
Dopamine and Stress
Stress can either suppress or magnify a painful condition. Exposure to stress activates dopamine transmission in mesocorticolimbic dopamine neurons (including the nucleus accumbens and prefrontal cortex) and involves opioid mechanisms in the VTA, suggesting that the endogenous release of opioids in the VTA might be how stress inhibits tonic pain.
Chronic stress, however, can magnify pain through mechanisms including depletion of dopamine in the reward circuits. Furthermore, if an individual has genetic variants that predispose to reward deficiency syndrome including the DRD2A1 variant, there is a compromise in their ability to cope with stress, leading to further magnification of pain.
New Frontiers in the Understanding of Chronic Pain
Two arenas of recent scientific study of pain are evolving in ways that promise to revolutionize both our understanding and our treatment of chronic pain. These fields of study are neuroinflammation including the role of glial cells and the field of epigenetics.
Neuroinflammation and Glial Cells
Glial cells are cells found in the central and peripheral nervous system. They function to maintain balance in nerve and neurotransmitter activity, they form myelin (the coating of some nerve cells), and provide support and protection for neurons (nerve cells). Glial cells are derived from the immune system, the most common of which are microglia cells and astrocytes. Glia cells provide a supportive matrix for nerve cells, supplying nutrients and oxygen and aid in the repair of damaged cells. However, when activated, glial cells also are important in the evolution and maintenance of chronic nerve pain through the release of peptides known as cytokines that are pro-inflammatory, triggering chronic pain. They may play a role in opioid function including opioid-induced hyperalgesia and opioid tolerance. It is believed that pathologic glial cell activation plays a significant role in the evolution of fibromyalgia pain, central sensitization and other chronic pain syndromes.
Early studies suggest that medications or supplements that inhibit glial activation may reduce the development of chronic nerve pain or reduce the severity of existing nerve pain. They may also be useful in suppressing the development of opioid tolerance. Various inhibitors of glial activation that are being evaluated for clinical use in the managment of chronic pain include minocycline, a tetracycline-class antibiotic, low dose naltrexone, palmitoylethanolamide (PEA) and Acetyl-L-carnitine. There is some evidence as well that gabapentin may inhibit glial cell activation as another mechanism of action responsible for its effectiveness in treating nerve pain. Furthermore, there may be a role for antioxidants and NRF2 activators as well in the management of chronic nerve pain related to glial cell activation.
See: Neuroinflammation
Epigenetics
Over the last few decades our knowledge of genetics and the structure of DNA has dramatically advanced our knowledge in the field of health. Mapping the human genome probably represents the greatest scientific achievement of our lifetime with the promise to impact our understanding of health-related topics in ways never possible before. How genes get turned on or off, what makes them active or not and the nature of these processes are what make up the field of epigenetics. Within the broader field of epigenetics lies the field of neuroepigenetics that represents the study of the nervous system, the brain, nerve cells and that includes the study of pain. </s pan>
In the scientific community, a long standing debate has persisted regarding the role of “nature vs. nurture,” or is some cause and effect due to genetic or environmental influence – or both. It is now understood that these two elements are intimately intertwined so that environmental influences can impact the function of DNA and genes, and this impact can not only become permanent but can be passed on from one generation to the next. The implication of this is huge and has already triggered an incredible amount of research in this field of epigenetics. While this field of study is in its infancy, it is beginning to impact what we know about pain, the evolution from acute to chronic pain, the effects of chronic pain on the nervous system as well as new ideas how to manage pain.
This topic will be explored further in the near future. See below for references.
References:
- Introduction to Pain Pathways and Mechanisms
- Pain and the Neuromatrix in the Brain 2001
- Neuropathic pain – mechanisms and their clinical implications – 2014
- Hypothesizing that brain reward circuitry genes are genetic antecedents of pain sensitivity and critical diagnostic and pharmacogenomic treatment targets for chronic pain conditions – 2009
Pain – Descending Pathways
- Gabapentin loses efficacy over time after nerve injury in rats – Role of glutamate transporter-1 in the locus coeruleus – 2016
- Advances in Pain Research – Mechanisms and Modulation of Chronic Pain – 2018 Book
- Gabapentin Reverses Central Hypersensitivity and Suppresses Medial Prefrontal Cortical Glucose Metabolism in Rats with Neuropathic Pain – 2014
- Analgesic mechanisms of gabapentinoids and effectsin experimental pain models – a narrative review – 2018
- Strategies to Treat Chronic Pain and Strengthen Impaired Descending Noradrenergic Inhibitory System – 2019
- Descending Noradrenergic Inhibition An Important Mechanism of Gabapentin Analgesia in Neuropathic Pain – PubMed – 2018
- The link between chronic pain and Alzheimer’s disease – 2019
- The Noradrenergic Locus Coeruleus as a Chronic Pain Generator – 2017
Pain – Epigenetics
- the-emerging-field-of-neuroepigenetics-2013
- epigenetic-mechanisms-of-chronic-pain-2015
- epigenetic-regulation-of-chronic-pain-2015
- epigenetic-regulation-of-persistent-pain-2015
- targeting-epigenetic-mechanisms-for-chronic-pain-a-valid-approach-for-the-development-of-novel-therapeutics-pubmed-ncbi
- targeting-epigenetic-mechanisms-for-pain-relief-pubmed-ncbi
- telomeres-and-epigenetics-potential-relevance-to-chronic-pain-2012
- could-targeting-epigenetic-processes-relieve-chronic-pain-states-pubmed-ncbi
Pain – Epigenetics, Acute Pain Transition to Chronic Pain
- epigenetics-and-the-transition-from-acute-to-chronic-pain-2012
- epigenetics-in-the-perioperative-period-2015
- epigenetics-of-chronic-pain-after-thoracic-surgery-pubmed-ncbi
Pain – Epigenetics, Opioids
- chronic-opioid-use-is-associated-with-increased-dna-methylation-correlating-with-increased-clinical-pain-pubmed-ncbi
- epigenetic-regulation-of-opioid-induced-hyperalgesia-dependence-and-tolerance-in-mice-2013
- epigenetic-regulation-of-spinal-cord-gene-expression-controls-opioid-induced-hyperalgesia-2014-no-highlights
Pain – Neuroinflammation and Glial Cells
- evidence-for-brain-glial-activation-in-chronic-pain-patients-2015
- importance-of-glial-activation-in-neuropathic-pain-pubmed-ncbi
- glial-contributions-to-visceral-pain-implications-f
or-disease-etiology-and-the-female-predominance-of-persistent-pain-2016 - evidence-of-different-mediators-of-central-inflammation-in-dysfunctional-and-inflammatory-pain-interleukin-8-in-fibromyalgia-and-interleukin-1-neuroinflammation-rheumatoid-arthritis-2015
Emphasis on Education
Accurate Clinic promotes patient education as the foundation of it’s medical care. In Dr. Ehlenberger’s integrative approach to patient care, including conventional and complementary and alternative medical (CAM) treatments, he may encourage or provide advice about the use of supplements. However, the specifics of choice of supplement, dosing and duration of treatment should be individualized through discussion with Dr. Ehlenberger. The following information and reference articles are presented to provide the reader with some of the latest research to facilitate evidence-based, informed decisions regarding the use of conventional as well as CAM treatments.
For medical-legal reasons, access to these links is limited to patients enrolled in an Accurate Clinic medical program.
Should you wish more information regarding any of the subjects listed – or not listed – here, please contact Dr. Ehlenberger. He has literally thousands of published articles to share on hundreds of topics associated with pain management, weight loss, nutrition, addiction recovery and emergency medicine. It would take years for you to read them, as it did him.
For more information, please contact Accurate Clinic.
Supplements recommended by Dr. Ehlenberger may be purchased commercially online or at Accurate Clinic.
Please read about our statement regarding the sale of products recommended by Dr. Ehlenberger.
Accurate Supplement Prices
.