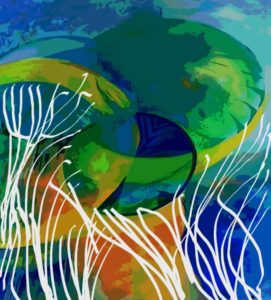
Jermane Krshnan
Inflammation
Resolving Neuroinflammation
Neuroinflammation is inflammation of nervous tissue. It may occur in response to a variety of triggers including trauma, infection, toxins, or auto-immune processes.
Neuroinflammation plays a central role in chronic pain but also in disorders such as fibromyalgia, depression, PTSD, multiple sclerosis, Parkinson’s Disease, Alzheimer Disease, brain and spinal cord injuries including chronic traumatic encephalopathy (CTE), stroke and schizophrenia.
The resolution of neuroinflammation has previously been considered a passive process but recent reseach offers means of reducing, perhaps resolving neuroinflammation.
Working on this page… come back soon
See:
- Neuroinflammation
- Systemic Inflammation
- Central Sensitization
- Oxidative Stress, Pain and Disease
- Antioxidants and Oxidative Stress
- Mitochondrial Dysfunction
See related topics:
- Pain
- Neurobiology of Pain
- Neuropathic (Nerve) Pain
- Neurobiology of Opioids
- Opioids
- Opioid Tolerances
- Medications for Pain
- Gabapentin (Neurontin) & Pregabalin (Lyrica)
- Toll-Like Receptor Antagonists (TLR-4)
- Traumatic Brain Injury
Key to Links:
- Grey text – handout
- Red text – another page on this website
- Blue text – Journal publication
Definitions and Terms Related to Pain
Resolving Neuroinflammation
Understanding how to treat neuroinflammation requires understanding the process of inflammation. Inflammation is part of the body’s defense mechanism in which the immune system recognizes threat in the form of injury, irritation or infection and removes harmful and foreign compounds as part of the healing process. Inflammation can be either acute or chronic, and it can also be either localized or systemic (widely distributed). Inflammation can be associated with changes in metabolism and may impact the hormonal or nervous systems.
There is little distinction between neuroinflammation and systemic inflammation (SI). Systemic inflammation occurs when the body’s immune system is constantly being stimulated as a result of stress, infection, chronic pain or chronic disease. Persistence of SI can lead to increased susceptibility to infections and to increased increased risk of diseases associated with aging.
Treatmen of neuroinflammation cannot be separated from the treatment of systemic inflammation, including addressing risk factors and behavioral and lifestyle modification. See: Systemic Inflammation
The process of neuroinflammation is complex and the methods to treat it are equally complex. The factors that contribute to neuroinflammation that offer potential targets for treatment include:
- Microglia activation of pro-inflammatory mediators
- Oligodendrocytes
- Microbiome and the Integrity of the blood-brain barrier (BBB) and the Intestinal Epithelial Barrier (IEB)
- Mediators with the Capacity to Actively Resolve Inflammation
- The Locus Coeruleus (LC) and Noradrenaline (norepinephrine – NE) System
- Mast Cell Activation
- Epigenetics of Neuroinflammation
- The Mesolimbic Dopamine (DA) system
1. Microglia activation of pro-inflammatory mediators
Glial cells are cells found in the central and peripheral nervous system. They function to maintain balance in nerve and neurotransmitter activity and provide support and protection for neurons (nerve cells). Glial cells are derived from the immune system, the most common of which are microglia cells and astrocytes.
When activated, glial cells are important in the evolution of neuroinflammation and chronic nerve pain through the release of peptides known as pro-inflammatory cytokines. In healthy brains, inflammatory processes normally cease and microglia transition back to an anti-inflammatory state. Sustained activation of pro-inflammatory microglia leads to chronic neuroinflammation characterized by high concentrations of neurotoxic compounds the brain. This failure to resolve the pro-inflammatory processes is a characteristic of many neurological disorders.
Following activation, glia cells release pro-inflammatory cytokines/chemokines including:
-
- Tumor necrosis factor (TNF)
- Interleukin-1beta (IL-1β)
- Interleukin-6 (IL-6)
- Interleukin-8 (IL-8)
- Chemokine (C–C motif) ligand 2 (CCL-2), also known as monocyte chemoattractant protein 1 (MCP-1)
- Brain-derived neurotrophic factor (BDNF)
- Nerve growth factor (NGF)
- Glutamate
- Substance P (SP)
Treatment Directed at Inhibiting Microglial Activation
Opioids and medications such as gabapentin (Neurontin), pregabalin (Lyrica) and duloxetine (Cymbalta) have been the conventional approaches to reduce chronic pain by their action on transduction and transmission of electrical impulses in neurons. Unfortunately, this conventional approach provides only limited success in controlling chronic pain and its progression.
In part, the reason for this failure is that this “nerve-based” approach fails to address the fact that the initiation and maintenance of nerve pain (or “neuropathic pain”) largely depends on neuroinflammation. Early studies suggest that medications or supplements that reduce neuroinflammation by inhibiting glial activation and/or stabilizing mast cells may reduce the development of chronic nerve pain or reduce the severity of existing nerve pain. They may also be useful in suppressing the development of opioid tolerance.
Various inhibitors of glial activation that are being evaluated for clinical use in the managment of chronic pain include minocycline, a tetracycline-class antibiotic, low dose naltrexone, palmitoylethanolamide (PEA) and Acetyl-L-carnitine. There is some evidence as well that gabapentin may inhibit glial cell activation as another mechanism of action responsible for its effectiveness in treating nerve pain. Furthermore, there may be a role for antioxidants and NRF2 activators in the management of glial cell activation.
At this time, the following agents are considered good candidates for treating neuroinflammation through stabilization and/or suppression of glial cells (and mast cells):
-
- Palmitoylethanolamide (PEA)
- Cannabidiol (CBD)
- Gabapentin
- Acetyl-L-carnitine
- Antioxidants
- NRF2 activators
- Minocycline – Recognized as a microglia inhibitor
- Low-dose naltrexone
- DHEA – Early/weak evidence for benefit in depression and regulation of the blood-brain barrier
2. Oligodendrocytes
Oligodendrocytes, the myelin-producing cells of the central nervous system (CNS), may also participate in the pain process. In addition to their production of myelin, oligodendrocytes support nerve function and long-term integrity. Oligodendrocyte damage/dysfunction leads to spinal nerve axon pathology and the induction/maintenance of increased pain sensitivity. Also, like glial cells and mast cells, they produce and respond to chemokines/cytokines that modulate CNS immune responses and interact with microglia. In the case of multiple sclerosis (MS), for example, autoimmune inflammation driven by invading peripheral immune cells leads to injury/degeneration of oligodendrocytes and neurons, and contributes to the neuropathic pain often experienced by MS patients.
3. Microbiome and the Integrity of the blood-brain barrier (BBB) and the Intestinal Epithelial Barrier (IEB)
In normal physiological conditions, the blood-brain barrier (BBB) prevents entry of most drugs, chemicals, toxins and peripheral blood cells into the brain and central nervous system. The BBB is an extensive network of endothelial cells (ECs) in brain capillaries together with neurons and glial cells, including microglia, that form a neurovascular unit (NVU). The communication between these cells maintains a proper environment for brain function.
The integrity of the BBB, which prevents”inappropriate” molecules from entering the central nervous system and brain, is dependent on the maintenance of “tight junctions,” where the cells of the blood vessel interface with adjoining cells. Changes in the interactions between blood vessel endothelium and microglia are associated with a variety of inflammation-related diseases in which BBB permeability is compromised. Evidence indicates that activated microglia modulate expression of tight junctions, which are essential for BBB integrity and function. On the other hand, the endothelium can in turn regulate the state of microglial activation.
Trauma and its associated stress induces a local inflammatory response causing disruption and dysfunction of the BBB increasing its permeability. This results in the infiltration of peripheral immune and inflammatory cells such as neutrophils, monocytes, mast cells (see below), and T cells into the brain. These cells become “activated,” immediately releasing inflammatory proteins called cytokines and chemokines within hours post-injury. These mast cell-derived inflammatory mediators further increase blood brain barrier (BBB) permeability and activate localized brain-based immune glial cells such as microglia and astrocytes (see below).
When activated, microglia and astrocytes increase production of similar inflammatory cytokines. Furthermore, all of these inflammatory mediators increase vascular permeability and increase escape and recruitment of immune and inflammatory cells at the site of injury. When the integrity of the BBB is compromised through inflammation or injury, there is increased permeability of the BBB, allowing for increased introduction of inflammatory chemicals, drugs and toxins to enter the central nervous system (CNS) – spinal cord and brain.
Although loss of BBB integrity is associated with several neuropathological disorders including depression, treatments that improve or stabilise the BBB are scarce. At this time, one focus of treatment of impaired BBB integrity lies in the stabilization of glial cells and mast cells. A 2017 study suggests that dehydroepiandrosterone sulfate (DHEAS) supports the integrity of the BBB. DHEA has shown evidence for benefit in the treatment of depression. Palmitoylethanolamide (PEA) has also demonstrated evidence that it glial cells and mast cells.
The Blood Brain Barrier and the Intestinal Epithelial Barrier (IEB)
A growing body of evidence demonstrates that the integrity of the BBB is linked to the integrity of the intestinal epithelial barrier (IEB), the analogous structure to the BBB in the gut. In turn, the integrity of the IEB is linked to the gut microbiome, the populati0n of microbes in the intestinal tract, Disruption of the IEB leads to a condition called “leaky gut syndrome,” in which a disruption of the tight junctions of the cells lining the gut wall allows for the pathologic invasion of compounds within the gut into the blood and systemic circulation.
These invasive compounds include bacterial products and dietary antigens which trigger an immune response causing the release of pro-inflammatory chemicals. This in turn contributes to the condition of systemic inflammation which is tied into neuroinflammation, and the potential development of a number of disease states.
The gut microbiome appears to be a significant factor contributing to the maintenance or the breakdown of the IEB. The gut microbiome is influenced and modified by a number of factors including stress and drugs, in particular antibiotics, NSAIDs and possibly opioids.
“Leaky Gut Syndrome” is believed to be associated with many pathological states, especially stress-related disorders. Leaky Gut Syndrome has been implicated in Irritable Bowel Syndrome (IBS), inflammatory bowel disease (Crohn’s and ulcerative colitis), fibromyalgia, depression, headaches and other chronic pain-related conditions.
Treatment directed at maintaining a healthy microbiome requires dietary intervention, in particular maintaining an anti-inflammatory diet such as the Mediterranean diet, and avoidance of pro-inflammatory foods including highly processed foods. There is a role for dietary supplementation of prebiotics and probiotics.
See: Leaky Gut (Coming soon… )
4. Mediators with the capacity to actively resolve inflammation (resolvins, protectins & maresins)
Recent research has identified mediators with the capacity to actively resolve inflammation, endogenous agents called resolvins, protectins & maresins, that are involved with the process of shutting down neuroinflammation. It is hoped that in the future the means of harnessing these agents for therapeutic purposes will become available.
What is believed at this time, however, is that production of these agents may be promoted by low dose aspirin and omega-3 essential fatty acids while NSAIDs may inhibit their production.
5. The Locus Coeruleus (LC) and Noradrenaline (norepinephrine – NE) System
Increasing noradrenaline (norepinephrine – NE) may help facilitate anti- inflammatory functions of microglia. NE may also be neuroprotective by reducing of intracellular oxidative stress as well as reducing chronic pain, another contributor to neuroinflammation.
The main brain areas involved in descending pain modulation by direct noradrenergic projections to the spinal cord are the periaqueductal grey (PAG) and the locus coeruleus (LC). The PAG conveys input from cortical and subcortical regions and plays a key role in top–down pain modulation through relays in noradrenergic LC neurons, along with the coordination of noradrenaline (NA)-mediated attention, arousal and cognition. Disturbances of the NA-mediated actions of the LC have been associated with several neurodegenerative and psychiatric disorders.
6. Mast Cell Activation
Glial cells participate in inflammation not only directly, but also in to response to molecular mediators produced by other immune system-derived cells, both blood-borne (dendritic cells, lymphocytes, neutrophils), and tissue-resident (mast cells). Mast cell are an important signaling link between the peripheral immune system and the brain in an inflammatory setting. Due to their widespread tissue presence near blood vessels and surfaces exposed to the environment, mast cells function as environmental “sensors” to communicate physiological and/or immune responses. Mast cells detect and respond to changes in environmental temperature and barometric pressure and are believed to play a role in the increased perception of pain associated with changes in weather.
Mast cells are manufactured in the bone marrow and enter the circulation and then into peripheral tissues including connective tissue cells and mucosal cells. They maintain broad tissue distribution, often close to blood vessels and near boundaries between the body’s external environment and the internal milieu, such as skin, mucosa of lungs and digestive tract, and in mouth, eye conjunctiva, and nose. Mast cells also found in the nervous system, including meningeal tissures that surround the brain, brain tissue, and nerve sleeves. They are integral in allergic reactions and anaphylactic shock, stress, mood disorders, inflammatory pain, chronic and neuropathic pain and acute and chronic neurodegenerative disorders.
Mast cells are found in tissues innervated by small caliber sensory nerve fibers (A-delta and C-fibers responsible for pain transmission that extend from the periphery to the spinal cord and brain), in meninges, and apposing cerebral blood vessels. Mast cell’s key role in the inflammatory process, when activated, is to rapidly release granules (degranulation) of bioactive chemicals, pro-inflammatory mediators such as cytokines and others into the surrounding tissues.
Degranulation is triggered by direct injury (physical or chemical), stimulation of immune receptors (such as IgE in allergies) or by activated complement proteins. More than 50 mediators are known and their expression by mast cells is complex and determined to a large extent by tissue location. Additionally, mast cell-derived chemoattractants recruit other immune cells including eosinophils, monocytes, and neutrophils, and can induce T cell activation, proliferation, and cytokine secretion.
It is clear that glial cells and mast cells play major roles in neuroinflammatiom through their release of chemically active protein mediators that impact tissues and stimulate pain, acutely and chronically. Current research is focusing on medications and other agents that can stabilize glial cells and mast cells, suppress their release of mediators and thereby reduce the development and/or maintenance of chronic pain.
7. Epigenetics of Neuroinflammation
8. The Mesolimbic Dopamine (DA) System
The specifics of how chronic pain affects the mesolimbic dopamine (DA) system is not fully understood, but there is new evidence for a role of altered DA signaling, not only in the chronicity of pain, but also in its response to opioid drugs. This evidence suggests a mechanism in which chronic pain activates microglia in the VTA to inhibit DA transmission and disrupt reward behaviors by disrupting Cl homeostasis in GABAergic neurons. Inhibiting microglial activation restores normal DA signaling and reward behavior. Microglial activation is a critical component modulating reward behavior in chronic pain. Inhibiting microglial activation may be an effective strategy for restoring disrupted DA transmission in Reward Deficiency Syndrome (RDS) as well as disorders linked to RDS such as depression and addiction. Microglial inhibitors such as minocycline, palmitoylethanolamide (PEA) and others are being explored and demonstrating promise as effective agents in chronic pain.
See:
Genetic Testing: Reward Deficiency Syndrome
In normal physiological conditions, the blood-brain barrier (BBB) prevents entry of most drugs, chemicals, toxins and peripheral blood cells into the brain and central nervous system. Trauma and its associated stress induces a local inflammatory response causing disruption and dysfunction of the BBB increasing its permeability. This results in the infiltration of peripheral immune and inflammatory cells such as neutrophils, monocytes, mast cells, and T cells into the brain. These cells become “activated,” immediately releasing inflammatory proteins called cytokines and chemokines within hours post-injury. These mast cell-derived inflammatory mediators further increase blood brain barrier (BBB) permeability and activate localized brain-based immune glial cells such as microglia and astrocytes (See below). When activated, microglia and astrocytes increase production of similar inflammatory cytokines. Furthermore, all of these inflammatory mediators increase vascular permeability and increase escape and recruitment of immune and inflammatory cells at the site of injury.
Normal, optimal inflammatory responses and physiological levels of inflammatory mediators are beneficial and protect the body as they remove unwanted waste materials and repair damaged tissues. As such, the initial, acute inflammatory response is protective, and lipid mediators such as eicosanoids (prostaglandins and leukotrienes produced from the essential fatty acid arachidonic acid) play critical roles in the initial response, with interactions between prostaglandins, leukotrienes and pro-inflammatory cytokines amplifying inflammation.
Normally, these altered and reactive immune cells diminish their activity within 10–14 days after injury and the inflammatory response ceases. However, in some cases, this neuroinflammation continues and becomes chronic, leading to many of the manifestations of nerve pain, or “neuropathic” pain, such as hyperalgesia, allodynia and peripheral and central sensitization, all of which are characterized by a magnification of pain experience.
Neuroinflammation and Glial Cells
Neuroinflammation and Mast Cells
Future Areas of Research – Resolving Inflammation
The resolution of neuroinflammation has previously been considered a passive process. Recent research, however, has identified mediators with the capacity to actively resolve inflammation, endogenous agents called resolvins, protectins & maresins, that are involved with the process of shutting down neuroinflammation. It is hoped that in the future the means of harnessing these agents for therapeutic purposes will become available. What is believed at this time, however, is that production of these agents may be promoted by low dose aspirin and omega-3 essential fatty acids while NSAIDs may inhibit their production.
NMDA Receptors
Besides the opioid receptors, another receptor that plays a significant roles in pain, especially nerve pain, is the NMDA receptor. It remains uncertain how NMDA and opioid receptors interact but blocking the NMDA receptor appears to reduce the development of opioid tolerance and opioid induced hyperalgesia. The list of receptors important in pain is not limited to only these few receptors but these are the receptors that when understood can provide insights towards better choices for treating pain. Thus, understanding the nature of a person’s pain and understanding the difference in how medications work allows for tailoring an individualized pain management plan to optimize pain control. (For more information about opioid and NMDA receptors, see Neurobiology of Opioids).
Glia Cells and the Mesolimbic Dopamine System
The specifics of how chronic pain affects the mesolimbic dopamine (DA) system is not fully understood, but there is new evidence for a role of altered DA signaling, not only in the chronicity of pain, but also in its response to opioid drugs. This evidence suggests a mechanism in which chronic pain activates microglia in the VTA to inhibit DA transmission and disrupt reward behaviors by disrupting Cl homeostasis in GABAergic neurons. Inhibiting microglial activation restores normal DA signaling and reward behavior. Microglial activation is a critical component modulating reward behavior in chronic pain. Inhibiting microglial activation may be an effective strategy for restoring disrupted DA transmission in Reward Deficiency Syndrome (RDS) as well as disorders linked to RDS such as depression and addiction. Microglial inhibitors such as minocycline, palmitoylethanolamide (PEA) and others are being explored and demonstrating promise as effective agents in chronic pain.
See:
New Frontiers in the Understanding of Neuroinflammation and Chronic Pain
Two arenas of recent scientific study of pain are evolving in ways that promise to revolutionize both our understanding and our treatment of chronic pain. These fields of study are neuroinflammation including the role of glial cells and the field of epigenetics.
Neuroinflammation and Glial Cells
Glial cells are cells found in the central and peripheral nervous system. They function to maintain balance in nerve and neurotransmitter activity, they form myelin (the coating of some nerve cells), and provide support and protection for neurons (nerve cells). Glial cells are derived from the immune system, the most common of which are microglia cells and astrocytes. Glia cells provide a supportive matrix for nerve cells, supplying nutrients and oxygen and aid in the repair of damaged cells. However, when activated, glial cells also are important in the evolution and maintenance of chronic nerve pain through the release of peptides known as cytokines that are pro-inflammatory, triggering chronic pain. They may play a role in opioid function including opioid-induced hyperalgesia and opioid tolerance. It is believed that pathologic glial cell activation plays a significant role in the evolution of fibromyalgia pain, central sensitization and other chronic pain syndromes.
Early studies suggest that medications or supplements that inhibit glial activation may reduce the development of chronic nerve pain or reduce the severity of existing nerve pain. They may also be useful in suppressing the development of opioid tolerance. Various inhibitors of glial activation that are being evaluated for clinical use in the managment of chronic pain include minocycline, a tetracycline-class antibiotic, low dose naltrexone, palmitoylethanolamide (PEA) and Acetyl-L-carnitine. There is some evidence as well that gabapentin may inhibit glial cell activation as another mechanism of action responsible for its effectiveness in treating nerve pain. Furthermore, there may be a role for antioxidants and NRF2 activators as well in the management of chronic nerve pain related to glial cell activation.
The Endocannabinoid System and Neuroinflammation
The endocannabinoid system is comprised of cannabinoid type 1 (CB1) and type 2 (CB2) receptors, the endogenous cannabinoids anandamide (AEA) and 2-arachidonylglycerol (2-AG), as well as enzymes that regulate their production. Anandamide, the first endocannabinoid to be identified, binds CB1 receptors as well as CB2 receptors with relatively low affinity. The second endocannabinoid to be identified, 2- arachidonoylglycerol (2-AG) also activates both CB1 and CB2 receptors.
2-AG is up to 100- times more abundant than AEA. It is synthesized by diacylglycerol lipase (DAGL) and degraded primarily by monoacylglycerol lipase (MAGL) as well as alpha/beta-hydrolase domain (ABHD) containing enzymes such as FAAH, ABHD6 and ABHD12.
Although both of these endocannabinoids have effects on pain, AEA has greater effects on depression and anxiety whereas 2-AG appears to contribute more to the effects on movement and temperature regulation. When both endocannabinoids are elevated through dual blockade of FAAH and MAGL, their effects mimic that of Δ9- tetrahydrocannabinol (Δ9-THC) from Cannabis.
The endocannabinoid system is a promising therapeutic target for chronic neuroinflammation based on evidence that synthetic and endogenously produced cannabinoids modulate the pro-inflammatory response of microglia and may contribute to switch to an anti-inflammatory state.
Activation of cannabinoid type 2 (CB2) receptors appears to be the mechanism of action responsible for this switch. Many other components of the endocannabinoid system in microglia can also contribute to dynamic change in response to brain pathology.
Microglia possess the necessary components required to synthesize, degrade, and respond to extracellular endocannabinoids.
They can impact the ability of microglia to synthesize and degrade endocannabinoids or react to endogenous and exogenous cannabinoids.
Epigenetics
Over the last few decades our knowledge of genetics and the structure of DNA has dramatically advanced our knowledge in the field of health. Mapping the human genome probably represents the greatest scientific achievement of our lifetime with the promise to impact our understanding of health-related topics in ways never possible before. How genes get turned on or off, what makes them active or not and the nature of these processes are what make up the field of epigenetics. Within the broader field of epigenetics lies the field of neuroepigenetics that represents the study of the nervous system, the brain, nerve cells and that includes the study of pain.
In the scientific community, a long standing debate has persisted regarding the role of “nature vs. nurture,” or is some cause and effect due to genetic or environmental influence – or both. It is now understood that these two elements are intimately intertwined so that environmental influences can impact the function of DNA and genes, and this impact can not only become permanent but can be passed on from one generation to the next. The implication of this is huge and has already triggered an incredible amount of research in this field of epigenetics. While this field of study is in its infancy, it is beginning to impact what we know about pain, the evolution from acute to chronic pain, the effects of chronic pain on the nervous system as well as new ideas how to manage pain.
This topic will be explored further in the near future. See below for references.
References:
- Introduction to Pain Pathways and Mechanisms
- Pain and the Neuromatrix in the Brain 2001
- Neuropathic pain – mechanisms and their clinical implications – 2014
- Hypothesizing that brain reward circuitry genes are genetic antecedents of pain sensitivity and critical diagnostic and pharmacogenomic treatment targets for chronic pain conditions – 2009
Pain – Epigenetics
- the-emerging-field-of-neuroepigenetics-2013
- epigenetic-mechanisms-of-chronic-pain-2015
- epigenetic-regulation-of-chronic-pain-2015
- epigenetic-regulation-of-persistent-pain-2015
- targeting-epigenetic-mechanisms-for-chronic-pain-a-valid-approach-for-the-development-of-novel-therapeutics-pubmed-ncbi
- targeting-epigenetic-mechanisms-for-pain-relief-pubmed-ncbi
- telomeres-and-epigenetics-potential-relevance-to-chronic-pain-2012
- could-targeting-epigenetic-processes-relieve-chronic-pain-states-pubmed-ncbi
Pain – Epigenetics, Acute Pain Transition to Chronic Pain
- epigenetics-and-the-transition-from-acute-to-chronic-pain-2012
- epigenetics-in-the-perioperative-period-20
15 - epigenetics-of-chronic-pain-after-thoracic-surgery-pubmed-ncbi
Pain – Epigenetics, Opioids
- chronic-opioid-use-is-associated-with-increased-dna-methylation-correlating-with-increased-clinical-pain-pubmed-ncbi
- epigenetic-regulation-of-opioid-induced-hyperalgesia-dependence-and-tolerance-in-mice-2013
- epigenetic-regulation-of-spinal-cord-gene-expression-controls-opioid-induced-hyperalgesia-2014-no-highlights
Pain – Neuroinflammation and Glial Cells
- evidence-for-brain-glial-activation-in-chronic-pain-patients-2015
- importance-of-glial-activation-in-neuropathic-pain-pubmed-ncbi
- glial-contributions-to-visceral-pain-implications-for-disease-etiology-and-the-female-predominance-of-persistent-pain-2016evidence-of-different-mediators-of-central-inflammation-in-dysfunctional-and-inflammatory-pain-interleukin-8-in-fibromyalgia-and-interleukin-1-%ce%b2-in-rheumatoid-arthritis-2015
Resolving Inflammation
- Vagus nerve controls resolution and pro-resolving mediators of inflammation – 2014
- The Resolution Code of Acute Inflammation – Novel Pro-Resolving Lipid Mediators in Resolution – 2015
- Resolvins in inflammation: emergence of the pro-resolving superfamily of mediators. – PubMed – NCBI – 2018
- Resolvins and protectins – mediating solutions to inflammation – 2009
- Resolvins and inflammatory pain – 2011
- Resolution of inflammation – an integrated view – 2013
- Protectins and maresins – New pro-resolving families of mediators in acute inflammation and resolution bioactive metabolome – 2014
- Proresolving lipid mediators and mechanisms in the resolution of acute inflammation – 2014
- Novel Pro-Resolving Lipid Mediators in Inflammation Are Leads for Resolution Physiology – 2014
- Novel Anti-Inflammatory — Pro-Resolving Mediators and Their Receptors – 2011
- Lipid Mediators in the Resolution of Inflammation – 2015
- PPARγ activation ameliorates postoperative cognitive decline probably through suppressing hippocampal neuroinflammation in aged mice. – PubMed – NCBI – 2017
- Postoperative cognitive dysfunction in the aged: the collision of neuroinflammaging with perioperative neuroinflammation. – PubMed – NCBI – 2018
- The Role of Neuroinflammation in Postoperative Cognitive Dysfunction – Moving From Hypothesis to Treatment – 2018
- Treating inflammation and infection in the 21st century: new hints from decoding resolution mediators and mechanisms – 2017
- Structural Elucidation and Physiologic Functions of Specialized Pro-Resolving Mediators and Their Receptors – 2017
- LPS is a Switch for Inflammation in the Gut and Beyond
- Identification of specialized pro-resolving mediator clusters from healthy adults after intravenous low-dose endotoxin and omega-3 supplementation – a methodological validation – 2018
- The Protectin Family of Specialized Pro-resolving Mediators – Potent Immunoresolvents Enabling Innovative Approaches to Target Obesity and Diabetes – 2018
- Protectins and Maresins – New Pro-Resolving Families of Mediators in Acute Inflammation and Resolution Bioactive Metabolome – 2014
- Functional Metabolomics Reveals Novel Active Products in the DHA Metabolome – 2012
- A focused review on CB2 receptor-selective pharmacological properties and therapeutic potential of β-caryophyllene, a dietary cannabinoid – 2021
- The link between chronic pain and Alzheimer’s disease – 2019
- Pain Modulation from the Locus Coeruleus in a Model of Hydrocephalus: Searching for Oxidative Stress-Induced Noradrenergic Neuroprotection – 2022
Emphasis on Education
Accurate Clinic promotes patient education as the foundation of it’s medical care. In Dr. Ehlenberger’s integrative approach to patient care, including conventional and complementary and alternative medical (CAM) treatments, he may encourage or provide advice about the use of supplements. However, the specifics of choice of supplement, dosing and duration of treatment should be individualized through discussion with Dr. Ehlenberger. The following information and reference articles are presented to provide the reader with some of the latest research to facilitate evidence-based, informed decisions regarding the use of conventional as well as CAM treatments.
For medical-legal reasons, access to these links is limited to patients enrolled in an Accurate Clinic medical program.
Should you wish more information regarding any of the subjects listed – or not listed – here, please contact Dr. Ehlenberger. He has literally thousands of published articles to share on hundreds of topics associated with pain management, weight loss, nutrition, addiction recovery and emergency medicine. It would take years for you to read them, as it did him.
For more information, please contact Accurate Clinic.
Supplements recommended by Dr. Ehlenberger may be purchased commercially online.
Please read about our statement regarding the sale of products recommended by Dr. Ehlenberger.
.