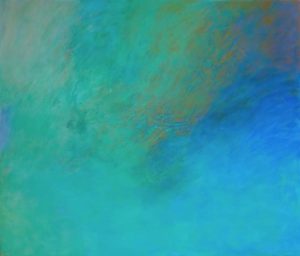
Jermane Krshnan “Only a fool thinks they are a wise man, a wise man knows he is a fool”
Inflammation
Systemic Inflammation
Systemic inflammation (SI) occurs when the body’s immune system is constantly being stimulated as a result of stress, infection, chronic pain or chronic disease. Persistence of systemic inflammation can lead to increased susceptibility to infections and to increased increased risk of diseases associated with aging including metabolic syndrome, which includes the triad of high blood pressure, high blood sugar and and elevated cholesterol (dyslipidemia). Chronic inflammatory diseases are the greatest threat to health and the most significant cause of death in the world.
See also:
- Pathophysiology of Chronic Inflammation
- Neuroinflammation
- Resolving Neuroinflammation
- Obesity
- Visceral Fat
- Oxidative Stress, Pain and Disease
- Antioxidants and Oxidative Stress
- Mitochondrial Dysfunction
- NRF2 Activators
- Nicotinamde Riboside (Niacel)
- Vitamins
- Vitamin C
- Vitamin E
The medical information on this site is provided as a resource for information only, and is not to be used or relied upon for any diagnostic or treatment purposes and is not intended to create any patient-physician relationship. Readers are advised to seek professional guidance regarding the diagnosis and treatment of their medical concerns.
Key to Links:
- Grey text – handout
- Red text – another page on this website
- Blue text – Journal publication
Systemic Inflammation
To understand system inflammation, it is important to put it in context with other aspects of inflammation, both acute and chronic and localized vs. systemic.
Inflammation
Inflammation is part of the body’s defense mechanism in which the immune system recognizes threat in the form of injury, irritation or infection and removes harmful and foreign compounds as part of the healing process. Inflammation can be either acute or chronic, and it can also be either localized or systemic (widely distributed). Inflammation can be associated with changes in metabolism and may impact the hormonal or nervous systems. Bio-behavioral effects of inflammation can include a number of “sickness symptoms.”
Some of the common signs and symptoms that develop during chronic inflammation:
- Malaise
- Fatigue
- Altered sleep
- Reduced appetite, weight gain or weight loss
- Reduced libido
- Sadness
- Impaired ability to experience pleasure (anhedonia)
- Depression and mood disorders
- Social-behavioral withdrawal
- Body pain, Joint and muscle
- Gastrointestinal symptoms: constipation, diarrhea, and acid reflux
- Frequent infections
Acute Inflammation
Tissue damage due to trauma, infection, or toxic compounds can induce acute inflammation. Acute inflammation starts quickly, may become severe in a short time and its symptoms may persist for days. A normal inflammatory response is characterized by time-restricted upregulation of inflammatory activity that occurs when a threat is present and then resolves once the threat resolves. Subacute inflammation is the period between acute and chronic inflammation and may last 2 to 6 weeks.
However, social, psychological, environmental and biological factors may contribute to the failure to resolve acute inflammation and, in turn, promote a state of low-grade, non-infectious systemic chronic inflammation which is characterized by the activation of immune components that are often distinct from those engaged during an acute immune response.
Chronic Inflammation
Chronic inflammation is also referred to as slow, long-term inflammation lasting for prolonged periods of several months to years. Generally, the extent and effects of chronic inflammation vary with the cause of the injury and the ability of the body to repair and overcome the damage.
Chronic inflammation can result from the following:
-
- Failure to eliminate an infection causing an acute inflammation such as bacteria, protozoa, fungi, and other parasites that resist host defenses and remain in the tissue for an extended period.
- Exposure to a low level of a particular irritant or foreign material that cannot be eliminated or broken down by the body including industrial substances that can be inhaled over a long period such as silica dust.
- An autoimmune disorder in which the immune system recognizes a normal part of the body as a foreign antigen and attacks healthy tissue, such as rheumatoid arthritis (RA) and systemic lupus erythematosus (SLE).
- An impairment in the process of resolving inflammation, leading to persistent or recurrent inflammation.
- Inflammatory and biochemical inducers are causing oxidative stress and mitochondrial dysfunction such as increased production of free radical molecules, advanced glycation end products (AGEs), uric acid
(urate) crystals, oxidized lipoproteins, homocysteine, and others.
Acute Localized Inflammation
Inflammation associated with an acute injury such as a sprained ankle most often remains localized and may be characterized by redness, swelling (edema) and pain or sometimes itching. As with an acute injury, an acute infection also generally remains localized such as the case with acute sinusitis or bronchitis.
Acute Systemic Inflammation
However, acute systemic infection is called sepsis, which can lead to systemic inflammation. Sepsis with one or more organ failures is considered severe sepsis, and when accompanied by unstable low blood pressure, it is called septic shock. In severe cases such as what occurs sometimes with COVID-19 infections, this systemic inflammation presents as an overwhelming and sometimes fatal inflammatory response termed “Systemic Inflammatory Response Syndrome (SIRS).” It is associated with a condition called “cytokine storm,” in which there is a massive inflammatory response that is so overwhelming that it severely compromises lung, heart and other organ functions leading possibly to irreversible end-organ dysfunction and even death.
See: COVID-19
Chronic Systemic Inflammation (SI)
Persistence of the inflammatory response from acute to chronic can lead to major alterations in the cellular physiology of all tissues and organs including the immune system, which can result in impaired immune function, leading to increased susceptibility to infections and tumors and poor responses to vaccines. Chronic systemic inflammation (SI) can lead to increased increased risk of the metabolic syndrome, which includes the triad of high blood pressure, high blood sugar and and elevated cholesterol (dyslipidemia). It also can cause an increased risk of:
-
- Fatty liver disease (NAFLD);
- Cardiovascular disease;
- Chronic kidney disease;
- Various types of cancer; depression;
- Neurodegenerative diseases (Alzheimers, Parkinsons)
- Autoimmune diseases;
- Osteoporosis;
- Loss of muscle mass
Systemic Inflammation and Pain
Local inflammation, acute and chronic, is clearly a major factor in many chronic pain syndromes, especially the different types of arthritis (osteoarthritis, Rheumatoid etc.) and inflammatory bowel diseases (Ulcerative Colitis, Crohns). But systemic inflammation is an extremely important factor in chronic pain also due to neuroinflammation.
Neuroinflammation
Persisting inflammation in the nervous system is responsible for the transitioning of acute to chronic pain and the maintenance of chronic pain, whereby the pain associated with an acute injury becomes a chronic, sometimes lifelong, condition.
Causes and Risk Factors for Systemic Inflammation
There are multiple contributing factors associated with systemic inflammation, some of which may be causative while others may contribute to risk. Research indicates that SI is multifactorial: a combination of both endogenous factors (produced or synthesized by the body) and non-endogenous (environmental) factors.
Aging
Systemic inflammation often increases over the age 30, as indicated by studies showing that older individuals have higher circulating levels of cytokines, chemokines and acute phase proteins, as well as greater expression of genes involved in inflammation. The age-associated increase in these inflammatory molecules, termed “inflammaging,” may be triggered by mitochondrial dysfunction or free radical accumulation over time along with other factors.
Systemic inflammation in older individuals is thought to be caused in part by a complex process called cellular senescence, which is characterized by an arrest of cell proliferation and increased secretion of pro-inflammatory cytokines, chemokines and other pro-inflammatory molecules from cells.
A large study, the PolSenior study, conducted on 4979 adults aged ≥65 years aged ≥65 years, demonstrated that the inflammatory biomarkers, Interleukin-6 (IL-6) and C-reactive protein (CRP), levels were elevated in an age-dependent manner. However, people with no history of cardiovascular disease (CVD), type 2 diabetes, cancer or stroke showed a small but significantly lower levels of these two biomarkers (IL-6 and CRP).
CRP levels in particular, appear to be critically linked to the development of cardiovascular diseases. CRP in turn is known to be regulated by IL-6 and tumor necrosis alpha (TNF-α). Large and sustained elevation of CRP levels are associated with a very high risk of CVD and mortality.
Senescence
In the process of aging, cells can permanently stop dividing but not die, referred to as replicative senescence. A telomere is a region of repetitive DNA sequences at the end of a chromosome and each time a cell divides, the telomeres become slightly shorter. Eventually, they become so short that the cell can no longer divide successfully, and the cell dies.
Senescence can also be induced prematurely by a variety of stressors, such as oxidative stress, radiation and others. Unlike replicative senescence, the stress-induced premature senescence is independent of the telomere length or the number of cell divisions. How senescent cells develop is not fully understood, but it is multifactorial: a combination of both endogenous factors (produced or synthesized by the body) and exogenous (environmental) factors. Over time, large numbers of old (or senescent) cells can build up in tissues throughout the body.
Cellular senescence plays a role in complex biological processes including development, tissue repair, aging and age-related disorders. It contributes to many chronic conditions and diseases, including insulin resistance, cardiovascular disease, pulmonary arterial hypertension, chronic obstructive pulmonary disorder (COPD), Alzheimer’s and Parkinson’s diseases, macular degeneration, osteoarthritis and cancer.
Senescence and Caloric Restriction
Research is ongoing that evaluates methods of slowing or reversing senescence. Caloric restriction and fasting have been suggested to counteract cellular aging and other aging-related pathologies to promote healthy aging. In particular, short-term fasting cycles have been shown to diminish cardiovascular and metabolic risk factors as well as to promote cell regeneration and boost immune systems.
Intermittent and periodic fasting are safe strategies to improve cellular aging and disease risk factors, while causing minor or no side effects. Intermittent fasting lasting from 12 to 48 hours and repeated every 1 to 7 days and periodic fasting lasting 2 to 7 days and repeated once or more per month are recommended forms of fasting. See: Diet & Fasting
Endogenous Factors
Among the known endogenous causes of this phenotype are DNA damage, dysfunctional telomeres (sequences of DNA that protect chromosomes), epigenomic disruption (epigenetic changes are changes in the way genes are switched on and off without changing the actual DNA), mitogen dysregulation (a mitogen is a small bioactive protein or peptide that induces a cell to begin or enhances cell division) and oxidative stress. Except for oxidative stress, the endogenous causes may be more difficult to modify.
Oxidative Stress
One of the consequences of persisting SI is the development of “oxidative stress.” Oxidative stress is an imbalance in the body of excessive “oxidants” (oxidizing or chemically active, agents, including free radicals obtained from the diet or produced by the body) and insufficient “antioxidants” (chemically active agents that are also obtained from the diet or produced by the body) and neutralize oxidants. This overabundance of oxidants causes damage to biomolecules, (lipids, proteins, DNA), cells and tissue, eventually contributing to aging and chronic diseases.
Chronic SI and oxidative stress are two pathophysiological conditions that coexist because they can mutually induce each other. Both SI and oxidative stress disrupt normal cellular physiology and they both contribute to the development and progression of chronic diseases including metabolic syndrome and diabetes, cardiovascular disease, cancers, neurodegenerative disorders, chronic kidney disease, liver disease, and rheumatoid arthritis.
See: Oxidative Stress, Pain and Disease
Non-Endogenous Factors
The non-endogenous contributors include chronic infections, lifestyle-induced obesity, microbiome imbalance, diet, social and cultural factors and environmental and industrial toxins. It is these non-endogenous contributors that allow for intervention as a means of reducing SI.
The differences in the extent to which older adults exhibit SI is thought to reflect inter-individual differences in exposure to the non-endogenous contributors although studies are limited. Nevertheless, differences in non-communicable diseases associated with SI exist across cultures and countries.
Dietary and Lifestyle Habits
Most importantly, SI-related diseases have increased significantly in young and old individuals living in industrialized countries maintaining a Western lifestyle but are relatively rare among individuals in non-Westernized cultures who maintain diets and lifestyles resembling those present during most of human evolution.
Populations of hunter-gatherers and other non-industrialized societies such as the Shuar hunter-gatherers of the Ecuadorian Amazon, Tsimané forager-horticulturalists of Bolivia, Hadza hunter-gatherers from Tanzania, subsistence agriculturalists from rural Ghana and traditional horticulturalists of Kitava (Papua New Guinea)—all of whom are minimally exposed to industrialized environments and have very low rates of inflammation-related chronic disease withy substantial fluctuations in inflammatory biomarkers that do not increase with age.
Furthermore, dietary and lifestyle habits, as well as exposure to a variety of different pollutants, can increase oxidative stress, up-regulate mitogenic signaling pathways and cause genomic and epigenomic changes that can induce the SI phenotype.
Evidence for the role of lifestyle in the development of chronic inflammation comes from a study of 210 healthy twins between 8 and 82 years old, which determined that non-heritable factors are the strongest contributors to differences in chronic inflammation between individuals and the main drivers of SI are exposure to environmental factors. These environmental exposures have been collectively called the exposome, referring to a person’s lifelong exposure to physical, chemical and biological elements, starting from the prenatal period onward.
Lifestyle, Social and Physical Environment
Individuals in the non-industrialized populations mentioned above have relatively short life expectancies so some die before showing signs of advanced aging. Their relative absence of SI-related diseases, however, has not been attributed to genetics or to having a shorter life expectancy, but instead due to lifestyle factors and their social and physical environments. Their lifestyles include higher levels of physical activity, diets of mainly fresh with minimally processed foods and less environmental pollutants. These individuals maintain circadian rhythms more closely synchronized with diurnal fluctuations in sunlight exposure and their social stressors are different from those typically present in industrialized societies.
While industrialization provides many benefits that contribute to increased average life expectancy, these changes also causes radical shifts in diet and lifestyle that are very different from the evolutionally shaped human physiology. This is believed to have created an evolutionary mismatch in humans that has been hypothesized to be a major cause of SI.
Stress and Sleep Disruption
Both physical and emotional stress are associated with inflammatory cytokine release. Stress can also disrupt sleep and individuals with irregular sleep schedules are more likely to have chronic inflammation than consistent sleepers sleep disorders are also considered as one of the independent risk factors for chronic inflammation.
Artificial Light, Blue Spectrum
A feature of modern lifestyle is increased exposure to artificial light, especially in the blue spectrum, often at unnatural biologic times. Exposure to blue light, especially after sundown, increases arousal and alertness at night and may disrupt circadian rhythms which in turn promotes inflammation. Night-shift work has been shown to increase risk for the metabolic syndrome, obesity, type 2 diabetes and cardiovascular diseases as well as in breast, ovarian, prostate, colorectal and pancreatic cancers.
Physical Activity
Industrialization has caused a significant overall decrease in physical activity, where in the United States approximately 50% of adults are considered physically inactive. Skeletal muscle is an endocrine organ that produces and releases cytokines and other small proteins, called myokines, into the blood during muscle contraction which reduce inflammation. Low physical activity has been found to directly increase levels of CRP and pro-inflammatory cytokine levels (biomarkers for SI, see below).
This increase in pro-inflammatory cytokines in individuals who are chronically inactive promotes several inflammation-related conditions, including insulin resistance, dyslipidemia, endothelial dysfunction, high blood pressure and loss of muscle mass (sarcopenia), that increase risk for cardiovascular disease, type 2 diabetes, fatty liver disease, osteoporosis, various cancers, depression, dementia and Alzheimer’s disease.
Obesity
In 2013, the American Medical Association declared obesity a disease, with more than the 150 million Americans that are over-weight or obese. While the classic definition of obesity is a body mass index (BMI) ≥30, obesity now includes normal-weight individuals with elevated body fat percentage or increased visceral fat and individuals with metabolic syndrome, characterized by elevated waist circumference, fasting blood sugar, triglycerides, and blood pressure and reduced HDL cholesterol. (See: Obesity)
Physical inactivity increases the risk for obesity and, in particular, increased visceral fat (intra-abdominal adipose tissue build up around organs, or VAT). People with increased visceral fat are at higher risk of atherosclerosis, insulin resistance, stroke, type 2 diabetes, and heart disease. (See: Visceral Fat)
VAT is not just fat cells. It is considered to be an active endocrine, immunological and metabolic body organ that is composed of various cells, including immune cells, such as macrophages. As such, excessive visceral fat is a major trigger of inflammation. It is reported that the BMI of an individual is proportional to the amount of pro-inflammatory cytokines secreted.
These VAT cells produce reactive oxygen species (such as free radicals – see: Oxidative Stress), and release of DAMPs (see below). These compounds further induce the secretion of numerous pro-inflammatory molecules, including adipokines, cytokines (such as IL-1β, IL-6, TNF- α), and chemokines by various VAT cells. These pro-inflammatory molecules in turn stimulate the infiltration of more various immune cells into the VAT, thereby amplifying inflammation, contributing to SI.
Furthermore, TNF-α and other molecules can cause adipocyte (fat cells) insulin resistance, which increases lipolysis (breakdown of fat), with the resulting spillover of lipids into other organs, such as the liver and pancreas, where they can contribute to fatty liver and pancreatic and liver insulin resistance and the development of diabetes.
In summary, visceral obesity increases risk for cardiometabolic, neurodegenerative and autoimmune diseases, as well as several cancers. In adults, it promote age-related disease risk, but obesity first emerges in childhood and may be playing a key role in promoting inflammation and age-related disease risk worldwide.
Obesity and Pain
Obesity is associated with increased pain and reduced benefit from treatments of pain. Individuals with obesity experience chronic pain at much higher rates than those of low or normal weight. Based on surveys, pain rates are 68% to 254% higher in individuals classified as obese compared with non-obese groups.
Obesity has a mechanical component that contributes to pain such as the additional weight causing compression of a joint. For example, it has been shown that just a reduction of 5% body weight can result in a noticeable reduction of chronic knee pain. It has also been shown that an 11-pound weight loss can be associated with a 50% reduction in the risk of symptomatic knee arthritis. In one study of knee arthritis, an 11% loss of body weight in patients with an average BMI of 35.9 resulted in a 50% decrease in pain over 8 weeks .
But pain that occurs with obesity is not simply mechanical, related to joint compression or spinal misalignment. Obesity-related pain is not found just in weight bearing joints and the lower back, but it also includes the thoracic spine, neck, upper extremities (including rotator cuff tendinitis and carpal tunnel syndrome) and hands, as well as headache and fibromyalgia. In a 6-month controlled weight loss study in obese patients with fibromyalgia, muscle pain was reduced accompanied by significantly improved quality of life.
Obesity-related pain can be directly attributed to systemic inflammation. Thus, interventions such as exercise that target both systemic inflammation and obesity are important in reducing pain as well. It should also be noted that while people with pain and obesity may feel discomfort initially as they begin an exercise program, post-exercise soreness does not equate with long-term worsening of their pain. Research shows that moderate exercise does not cause progression of osteoarthritis. A long-term study of 30,000 people also confirmed that exercise does not increase the risk of osteoarthritis at any level of BMI.
Microbiome Dysbiosis
It is believed that a complex balance exists in the intestinal ecosystem of microorganisms (microbiome) that, if imbalanced or dysregulated creating “dysbiosis,” can contribute to low-grade SI. Possible triggers of dysbiosis and its associated intestinal hyperpermeability, may include diet, the overuse of antibiotics, nonsteroidal anti-inflammatory drugs (NSAIDs) and proton-pump inhibitors (such as Prilosec).
Obesity, which is strongly linked to changes in the gut microbiome, may also lead to SI through gut microbiome-mediated mechanisms. These mechanisms include increased intestinal permeability allowing for increased absorption of toxins, activation of pattern recognition receptors, such as Toll-like receptors in immune cells and inflammation-mediated insulin resistance.
Zonulin, a protein that increases intestinal permeability, is elevated in obesity and in persons with type 2 diabetes, fatty liver disease, coronary heart disease, autoimmune diseases and cancer. Elevated blood zonulin levels are associated with SI and physical frailty.
Diet
Diets rich in saturated fat, trans-fats, or refined sugar are associated with higher production of pro-inflammatory molecules. The modern diet is relatively low in fruits, vegetables and other fiber- and prebiotic-rich foods and high in refined grains, alcohol and ultra-processed foods, particularly those containing emulsifiers. These dietary factors can imbalance the gut microbiota composition and impair function and these factors are linked to increased intestinal permeability and epigenetic changes in the immune system that contribute to low-grade SI.
Advanced Glycation and End-products (AGE) and Oxidative Stress
In addition to the influence of diet on inflammation, advanced glycation and lipoxidation end-products are also involved. These products, which are formed during the processing of foods or when foods are cooked at high temperatures and in low-humidity conditions, stimulate appetite and are linked to overeating, obesity and SI. Furthermore, high-glycemic-load foods, such as sugars and refined grains cause increased oxidative stress that activates inflammatory genes.
Salt
Other dietary components that contribute to inflammation include trans fatty acids and dietary salt. Salt has been shown to stimulate immune cells (macrophages) to release pro-inflammatory cytokines. In addition, high salt intake can induce changes in gut microbiota including reduced Lactobacillus population contributing to dysbiosis. Lactobacillus is critical for health because it enhances the integrity of the intestinal epithelial barrier, thus reducing SI.
Micronutrients
Several other nutritional factors also promote SI such as deficiencies in micronutrients, including zinc and magnesium, which are related to eating processed or refined foods that are low in vitamins and minerals.
Omega-3 Fatty Acids
Suboptimal intake of omega-3 from fish and other sources impairs the resolution phase of inflammation. Longchain omega-3 fatty acids—especially eicosapentaenoic acid (EPA) and docosahexaenoic acid (DHA) also modulate the expression of genes involved in metabolism and inflammation. More importantly, they are the body’s building blocks to molecules such as resolvins, maresins and protectins that are involved in the resolution of inflammation.
The main contributors to low omega-3 status is a low intake of fish and high intake of vegetable oils that are high in linoleic acid, which displaces omega-3 fatty acids in cell membrane phospholipids. Research has have shown that omega-3 fatty acid supplementation reduces inflammation and may thus have health-promoting effects.
Diet and Mortality
Evidence linking diet and mortality is strong. The National Center for Health Statistics in the United States showed that the dietary risk factors associated with the greatest mortality among American adults in 2005 were high dietary trans fatty acids, low dietary omega-3 fatty acids, and high dietary salt. In addition, a recent systematic analysis of dietary data from 195 different countries identified poor diet as the main risk factor for death in 2017, with excessive sodium intake being responsible for more than half of diet-related deaths.
Finally, when combined with low physical activity, eating hyperpalatable processed foods that are high in fat, sugar and salt can cause major changes in cell metabolism that lead to dysfunctional mitochondria and increased production (and defective disposal) of misplaced, misfolded and oxidized endogenous molecules.
These altered molecules, which increase with age, can be recognized as DAMPs (see below) by innate immune cells, which in turn amplify the inflammatory response and contribute to a biological state that has been called “inflammaging,” defined as the “the long-term result of the chronic physiological stimulation of the innate immune system.” Inflammaging involves changes in the brain, gut, liver, kidney, fat tissue and muscle which drive SI.
Environmental Factors and Toxins
Exposure to various environmental agents, including air pollutants, hazardous waste products and industrial chemicals promote SI. Each year, an estimated 2,000 new chemicals are introduced into items that people use or ingest daily, including foods, personal care products, prescription drugs, household cleaners, lawn care products and microplastics. Understanding the associated increase in the contribution of environmental chemicals to human disease is beyond this website, but see: https://ntp.niehs.nih.gov).
Numerous chemicals to which people are commonly exposed greatly alter molecular pathways that contribute to inflammation and inflammation-related disease risk. These chemicals include phthalates, per- and polyfluoroalkyl substances, bisphenols, polycyclic aromatic hydrocarbons and flame retardants.
These compounds and others promote inflammatory activity via multiple mechanisms. They can be toxic to cells, cause oxidative stress or act as disrupt hormone systems, starting in utero. These chemicals are suspected of playing a role in causing hormone- dependent cancers, metabolic syndrome, type 2 diabetes, hypertension, cardiovascular diseases, allergies, asthma, and autoimmune and neurodegenerative diseases. Tobacco use is associated with a variety of inflammation-related diseases. Cigarette smoking is associated with lowering of the production of anti-inflammatory molecules and with inducing inflammation.
Developmental Origins of Chronic Systemic Inflammation
Childhood
The development of SI can actually have roots in childhood where circumstances significantly impact metabolic and immune responses later in life, that promote SI in adulthood. Childhood obesity, for example, is strongly associated with major changes in fat tissue and metabolic dysfunction that cause metabolism-related-SI. Additionally, because obese children often become obese adults, the se pro-inflammatory conditions frequently persists into adulthood.
Also, there is evidence that exposure to psychological stress early in life, in the form of abuse, neglect, maltreatment, bullying or living in a low socioeconomic environment, can magnify nervous system responses to stress. This magnified esponses to stress upregulates inflammatory activity and impairs immune competence, leading to SI.
Prenatal Period
Even before childhood, the immune system is programmed during the prenatal period and is affected by epigenetic changes (changes associated with turning genes on or off) induced by maternal environmental exposures, including, infections, diet, psychological stress and drugs during intrauterine life. Even before conception, paternal factors may also have an epigenetic effects.
Together, these effects establish the potential for the transmission of risk for SI across generations. For example, maternal inflammation during pregnancy can pass an inflammatory ‘code’ through epigenetic modifications to their children, who will have increased risk for SI in childhood and adulthood. This means they will be more likely to develop inflammation-related health problems, including obesity, cardiovascular disease, certain cancer and neurological conditions like Alzheimers, among others. Furthermore these risks can continue to be passed on to future generations.
Chronic Systemic Inflammation and the Immune Response
Despite the heightened immune activity associated with SI generally increases with age, a majority of older adults have increased susceptibility to viral infections and weakened responses to vaccines. Elevated SI predicts reduced responsiveness to the hepatitis B vaccine and there is evidence that levels of the biomarker CRP are inversely correlated with one’s response to other vaccines, such as the herpes zoster and typhoid vaccines.
In summary, exposure to an inflammatory environment early in life is an important determinant of multiple aspects of an individual’s health and immunity in adulthood.
Assessing for Systemic Inflammation
Unfortunately, there are no highly effective laboratory tests to assess patients for chronic inflammation. Existing biomarkers for inflammation, mostly including CRP, IL-1β, IL-6 and TNF-α, demonstrate that inflammatory activity is related to disease and mortality risk. Given the enormous complexity of the inflammatory response, however, these biomarkers provide only limited information and don’t address anti-inflammatory regulatory pathways that may also be relevant for influencing inflammation-related disease risk.
Biomarkers for Chronic Systemic Inflammation
Despite evidence linking SI with disease risk and mortality, there are currently no universally accepted standard biomarkers for the presence of health-damaging chronic inflammation. Many studies use biomarkers of inflammation to understand or predict chronic disease and aging. Chronic low-grade inflammation seen with aging is commonly associated with pro-inflammatory biomarkers but most studies evaluate just one or a few biomarker at a time, leading to conflicting results due to complex interactions among biomarkers.
Studies have shown that some biomarkers of acute inflammation predict morbidity and mortality and may be used to assess age-related SI. This approach has limitations, however. For example, early work showed that monocyte levels of the inflammatory biomarkers IL-6 and IL-1Ra (but not IL-1β or TNF-α) increase with age >47. However, no difference in IL-1 and IL-6 expression has been found between young and older individuals when the health status of older individuals is strictly controlled.
A recent study examined levels of 18 inflammatory biomarkers in 41 healthy volunteers of different ages revealed that levels of IL-12 in women and C-reactive protein (CRP) in men were associated with older age, whereas no effects were found for IL-1β, IFN-α or TNF-α. Therefore, greater inflammatory activity is associated with older age, but not for all inflammatory markers and it is possible that these associations are due at least in part to increases in chronic ailments and frailty that are frequently associated with age rather than to biological aging itself.
Two blood tests that are inexpensive and good markers of systemic inflammation include high-sensitivity C-reactive protein (hsCRP) and fibrinogen.
C-Reactive Protein (CRP)
The blood level of CRP is a predictor of cardiovascular events in men and women. In a recent meta-analysis of data from more than 160,000 people across 54 long-term prospective studies, higher levels of circulating CRP were associated with a relative increase in risk for both coronary heart disease and cardiovascular disease and mortality.
Along similar lines, a recent study of more than 160,000 people from North Glasgow found that a combination of the inflammatory markers CRP (>10 mg/L), albumin (>35 mg/L) and neutrophil count (>7.5)) predicted all-cause mortality over 8 years, in addition to mortality due to cancer, cardiovascular and cerebrovascular disease.
The high-sensitivity C-reactive protein (hs-CRP) test is a more sensitive blood test that identifies lower levels of CRP when they are below a standard cut-off value for CRP. The hs-CRP can be used to find the risk for heart disease and stroke in people who don’t already have heart disease.To illustrate the difference between CRP and hs-CRP, standard testing measures CRP within the range of 10 to 1,000 mg/L, whereas hs-CRP values range from 0.5 to 10 mg/L. In simpler terms, hs-CRP measures trace amounts of CRP in the blood. The normal serum level for hsCRP is less than 0.55 mg/L in men and less than 1.0 mg/L in women.
Fibrinogen
The normal levels of fibrinogen are 200 to 300 mg/dl.
Inflammatory Cytokines
Measuring pro-inflammatory cytokines like tumor necrosis factor-alpha (TNF-alpha), interleukin-1 beta (IL-1beta), interleukin-6 (IL-6), and interleukin-8 (IL-8) are expensive but may identify specific factors causing chronic inflammation. However, these assays are not standardized like hs-CRP and fibrinogen.
Serum Amyloid A (SAA)
SAA (Serum Amyloid A) can also mark inflammation but is not a standardized test
Future Assessments of Chronic Systemic Inflammation
Recently, researchers developed a multidimensional approach to provide a more accurate interpretation of the various relationships between the biomarkers. This approach used principal component analysis (PCA) of 19 inflammatory biomarkers, with the first axis representing inflammatory activation (both pro- and anti-inflammatory markers) and a second axis representing innate immune response. The first but not the second axis was strongly correlated with age, and both were strongly predictive of mortality and multiple chronic diseases significantly predicted risk for multiple chronic diseases (cardiovascular disease, kidney disease and diabetes), in addition to mortality, but in opposite directions. Both axes were more predictive than any individual markers for baseline chronic diseases and mortality. Unfortunately, this technique is still a research tool not yet available to practicing clinicians.
More recently, another study evaluated a multi-omics approach to examine links between SI and disease risk. The researchers followed 135 adults conducting deep molecular profiling of participants’ whole-blood gene expression, termed the transcriptome; immune proteins—for example, cytokines and chemokines—termed the immunome; and cell subset frequencies, such as CD8+ T cell subsets, monocytes, natural killer (NK) cells, B cells and CD4+ T cell subsets.
This allowed the researchers to assess immune aging that describes an individuals’ immune functioning better than their chronological age, which accurately predicted all-cause mortality. This technique suggests potential future use for identifying at-risk patients in various clinical settings.
While these integrative, multi-level approaches for characterizing chronic SI are promising, this work is still very early and much more research is needed to identify and analyze SI-related biomarkers to determine the most useful information for quantifying age-related disease risk.
Biomarkers for (Acute) Systemic Inflammatory Response Syndrome
Systemic Inflammatory Response Syndrome (SIRS) is a serious condition in which there is inflammation throughout the whole body. It may be caused by a severe bacterial infection (sepsis), trauma, or pancreatitis but recently is the condition complicating COVID infection that is responsible for the high death rate of this disease. SIRS is characterized by fast heart rate, low blood pressure, low or high body temperature, and low or high white blood cell count.
There is good evidence that markers of the systemic inflammatory response, namely C-reactive protein and albumin have independent prognostic value in patients with a variety of cancers. Similarly, levels of neutrophils and lymphocytes (termed the neutrophil lymphocyte ratio, NLR) have also been shown to have independent prognostic value. Recently, a study evaluated these markers of the systemic inflammatory response, together with high sensitivity C-reactive protein measurements and platelet counts, and survival in more than 12,000 cancer patients.
This resulted in an optimised score (termed the optimised Glasgow Prognostic Score, oGPS) composed of high sensitivity C-reactive protein (>3mg/l), albumin (<35g/l), neutrophil (>7.5) and platelet (>400) counts that had a superior predictive value when compared with only C-reactive protein and albumin. It remains to be seen whether these markers and scores also are associated with survival in other disease states.
Mechanisms of Acute vs. Chronic Inflammation
Although they share some common mechanisms, the acute inflammatory response differs from the chronic, systemic response. The acute inflammatory response is typically initiated with infection via an interaction between pattern recognition receptors found on immune cells and structures on pathogen bacteria or viruses, called pathogen-associated molecular patterns (PAMPs). With resolution of infection and reduction of PAMPs, the immune system produces molecules such as lipoxins, resolvins, maresins and protectins that contribute to the resolution of inflammation.
An acute inflammatory response can also be activated by damage-associated molecular patterns (DAMPs) that are released in response to physical, chemical or metabolic injury or damage. In contrast to acute inflammation, systemic inflammation (SI) is often triggered by DAMPs in the absence of an acute infectious insult or activation of PAMPs. As such SI may persist at low levels and not resolve.
Persisting SI results in the immune cells producing continued higher circulating levels of cytokines (such as interleukin (IL)-1β and tumor necrosis factor (TNF)-α.), chemokines and acute phase proteins. These compounds maintain inflammation but also lead to collateral damage to tissues and organs over time. In addition, with SI, genes become activated that further result in the persistence of inflammation.
Interventions to Defend Against Systemic Inflammation
Despite the fact that the association between chronic SI and chronic disease is now widely recognized, research successfully targeting these risk factors and showing corresponding reductions in SI levels is limited.
Given the difficulty of doing research associated with experimentally manipulating variables such as diet, sleep and stress levels that affect inflammation, the majority of current studies have evaluated inflammatory biomarker data under baseline conditions in which the immune system is not challenged.
As such, current research provides little, if any, information regarding physiologic reactions to, or recovery from, infection, psychological or physiological stress associated with SI, particularly as related to therapeutic interventions. Additional research is required for understanding how individual interventions in inflammation-related disease risks impact the development of these diseases.
Many of the SI-promoting factors described above are at least partly modifiable—including physical inactivity, poor diet, night-time blue light exposure, tobacco smoking, environmental and industrial toxins exposure and psychological stress. Therefore the first steps in reducing SI and the risks for SI should be directed at these factors.
Additional steps that can be taken to avoid developing systemic inflammation:
Eat more anti-inflammatory foods:
Eating more natural foods including whole grains, plenty of vegetables and fruits such as avocados, cherries, kale, and fatty fish like salmon is helpful in avoiding inflammation. It is also important to avoid eating simple sugars, refined carbohydrates, high-glycemic foods, trans-fats, and hydrogenated oils.
Minimize intake of antibiotics and NSAIDs:
Antibiotics, antacids, and NSAIDs should be avoided when possible as they may disrupt the microbiome in the gut resulting in inflammation of the intestinal walls, causing “leaky gut,” which in turn promotes chronic, body-wide inflammation.
Treatment / Management of Chronic Systemic Inflammation
Lifestyle
Physical Exercise
Energy expenditure through exercise releases myokines that lower multiple pro-inflammatory molecules and cytokines, independently of weight loss.
Sleep
Sleep longer: Overnight sleep (ideally at least 7 to 8 hours) helps stimulating human growth hormones and testosterone in the body to rebuild itself.
Stress Less
Chronic psychological stress adds risk for losing the ability to regulate the inflammatory response and normal immune defenses. Yoga and meditation help alleviate stress-induced inflammation and its harmful effects on the body.
Weight Loss
Many dietary and lifestyle changes can be helpful in reducing chronic inflammation. Weight loss alone has been shown to be independently associated with clinically significant improvement in disease activity and inflammation in patients with arthritis for example. Weight loss also contributes in reducing many of the non-specific symptoms of chronic inflammation including joint and muscle pain, fatigue, depression, anxiety, mood disorders and gastrointestinal complications like constipation, diarrhea, and acid reflux.
Low-glycemic, Anti-inflammatory Diet
- Limit consumption of inflammation-promoting foods like sodas, refined carbohydrates, fructose corn syrup in a diet.
- Reduce intake of total, saturated fat and trans fats (dietary saturated and synthetic trans-fats aggravate inflammation). Processed and packaged foods that contain trans fats such as processed seed and vegetable oils, baked goods (like soybean and corn oil) should be reduced from the diet.
- Vegetables and low glycemic fruits: Blueberries, raspberries, blackberries, brussels sprouts, cabbage, broccoli, and cauliflower. These foods are high in natural antioxidants and polyphenols and other anti-inflammatory compounds. Cherries and cherry juice consumption has been shown to be uricosuric and inhibitory for IL-1 in patients with gout.
- Fiber: High intake of dietary soluble and insoluble fiber which lower levels of IL-6 and TNF- alpha as well as support a balanced microbiome.
- Nuts: such as pistachios, walnuts and almonds are associated with lowering the risk of cardiovascular disease and diabetes.
- Green and black tea polyphenols: Tea polyphenols are associated with a reduction in CRP. See: Green Tea
- Curcumin: turmeric, found in curcumin is a powerful antioxidant and anti-inflammatory associated with significant improvement in animal models of inflammatory diseases.
- Fish and fish oil: The richest source of the omega-3 fatty acids. High intake of omega-3 fatty acids is associated with lowering levels of TNF-alpha, CRP, and IL-6.
- Mung bean: Rich in flavonoids (particularly vitexin and isovitexin). It is a traditional food and herbal medicine known for its anti-inflammatory effects.
- Magnesium: Magnesium is one of the most anti-inflammatory dietary micronutrients, and its intake is associated with the lowering of CRP, IL-6, and TNF-alpha activity.
- Vitamin D: Vitamin D is anti-inflammatory by suppressing inflammatory mediators such as prostaglandins and nuclear factor kappa-light-chain-enhancer of activated B cells. See: Vitamin D
- Antioxidants: Vitamin E, zinc, and selenium.
- Sesame oil: A 2019 study evaluating supplementation with sesame oil plus 400 mg alpha-tocopherol/day demonstrated a beneficial effect for several cardiometabolic indices including serum total cholesterol, high-density lipoproteins, triglycerides, fibrinogen, insulin resistance, hs-CRP, systolic and diastolic blood pressure in patients with metabolic syndrome.
Cannabinoids:
THC, CBD and β-caryophyllene have potent anti-inflammatory activity See: Medical Marijuana – Introductory Principles
Conventional Drugs that Combat Chronic Inflammation
Metformin
Metformin is anti-inflammatory and commonly used to treat type II diabetes patients with dyslipidemia and low-grade inflammation. It reduces circulating TNF-alpha, IL-1beta, CRP, and fibrinogen levels.
Statins
Statins are anti-inflammatory and reduce multiple circulating and cellular mediators of inflammation. This effect appears to contribute in part to their reduction in cardiovascular events.
NSAIDs
Non-steroidal anti-inflammatory drugs (NSAIDs) like naproxen, ibuprofen, and aspirin acts by inhibiting an enzyme cyclooxygenase (COX) that contributes to inflammation. They are mostly used to relieve the pain caused by inflammation in patients with arthritis but should be used with caution under the direction of a physician due to their significant side effects of increased risk of gastrointestinal bleeding and ulcers, inflammation of the kidneys and increased risk for heart attacks and strokes. There are more effective and less potentially dangerous alternatives to NSAIDs.
Steroids
Corticosteroids offer several mechanisms to reduce inflammation and are prescribed for severe inflammatory conditions such as rheumatoid and psoriatic arthritis, systemic lupus, sarcoidosis, and asthma. however they are also associated with significant side effects including immune suppression.
The Role of Herbal and Other Supplements in Combating Systemic Inflammation
While there remains much to learn about how to reduce systemic inflammation (SI) on a molecular level, there are a number of compounds with evidence for benefit in inflammation. The mechanisms by which these compounds work are varied and likely synergistic in nature. Some impact the pathways involving the manufacture, release and effects of pro-inflammatory molecules such as cytokines, others work as antioxidants or stimulate cells to manufacture antioxidants. Some compounds work within the endocannabinoid system to reduce inflammation. The following is a list of agents that are believed to potentially suppress the development of SI, reduce existing SI or otherwise protect against SI.
Anti-inflammatories
Herbal supplements like ginger, turmeric, cannabis, hyssop, and Devil’s Claw (Harpagophytum procumbens, a traditional South African herbal remedy used for rheumatic conditions) have been shown to have anti-inflammatory properties. However, one should always consult with a doctor before their use and caution should be taken for using some herbs like hyssop and cannabis.
Curcumin: turmeric, found in curcumin, is a powerful antioxidant and anti-inflammatory associated with significant improvement in animal models of inflammatory diseases. See: Curcumin
Cannabinoids: THC, CBD and β-caryophyllene have potent anti-inflammatory activity. See: Medical Marijuana – Introductory Principles
Antioxidants
Antioxidants are produced in the mitochondria within cells throughout the body and serve to detoxify free radicals and protect tissues from such damage. Another important source of antioxidants is the diet, and diets rich in antioxidants are protective against the disease processes noted above. Fruits and vegetables are especially rich in antioxidants including: vitamin E, vitamin C, vitamin A, curcumin, resveratrol, glutathione, arginine, citrulline, taurine, creatine, selenium, zinc, and polyphenols found in tea.
Diets rich in fruits and vegetables result in high blood antioxidant capacity and reduced oxidative stress. Antioxidant activity is further supported by antioxidant enzymes, e.g. superoxide dismutase, catalase, glutathione reductase and glutathione peroxidase that exert synergistic actions in removing free radicals.
Individual Antioxidants:
-
- Vitamin E
- Curcumin
- Resveratrol
- N-Acetyl Cysteine (NAC)
- Alpha-Lipoic Acid
- Green Tea
- Milk Thistle (Silphos)
- Palmitoylethanolamide (PEA)
- Quercetin
- Vitamin C
- Coenzyme Q (CoQ-10)
Polyphenols
Polyphenols are a type of antioxidants that are commonly found in diets such as cranberries, red wine, green teas, and many others. Although the direct mechanisms of action of polyphenols are not fully understood, many studies have shown that polyphenols reduce the complications of obesity. Polyphenols has been shown to affect the gut microbiota and stimulate pathways that promote breakdown of fat tissue and lower body weight and fat mass by increasing fat use and energy expenditure. Polyphenol’s inhibitory actions include the downregulation of fat and triglyceride production and chronic obesity- related inflammation.
Polyphenols include:
Curcumin, a polyphenol antioxidant, has evidence for reducing insulin resistance, hyperglycemia, hyperlipidemia and other comorbidities associated with obesity. Evidence suggests curcumin can modulate various targets involved in obesity and metabolic diseases including suppression of inflammatory cytokines and lowering triglycerides and cholesterol.
Resveratrol is found in red grapes, mulberries and other fruits. It undergoes rapid metabolism in the small intestine and by the gut microbiota and has low plasma bioavailability. Resveratrol alters the composition of gut microbiota by increasing symbiotic bacteria like Bacteroidetes and decreasing opportunistic pathogens like Escherichia coli resulting in decreased levels of trimethylamine-N-oxide (TMAO), which is associated with obesity. Resveratrol increases breakdown of fat and decreases production of fat by the inhibition of PPARg and SIRT1. Resveratrol’s function as an antioxidant in redox cycling leads to the activation of Nuclear factor-erythroid 2-related factor-2 (Nrf2), increase in SOD, and reduction in BMI, blood pressure and body weight.
Epigallocatechin-3-gallate (EGCG) found in green tea is known for its anti-cancer effects, but studies have demonstrated a significant benefit in diabetes. In the context of T2DM, EGCG promotes glucose stabilization and inhibits fat and gluconeogenesis in the liver. EGCG improves the wound healing process through lowering of macrophage accumulation and inflammation.
Vitamin E
Vitamin E is known to have anti-oxidative, anti-inflammatory, anti-obesity, anti-hyperglycemic, anti-hypertensive and anti-hypercholesterolemic properties. As such it has been proposed as a supplement to offer benefits for these conditions, and particularly metabolic syndrome (MetS), a constellation of medical conditions including central obesity, hyperglycemia, hypertension, and dyslipidemia.
The best option in suppressing the progression of MetS is through a holistic approach, maintaining a healthy lifestyle, including exercise, reduction of stress and a healthy diet. The pathways regulated by vitamin E are critical in the development of MetS and its components, suggesting a supplemental pharmacological approach with vitamin E. The evidence provided by studies on the effects of vitamin E demonstrate the promising potential of vitamin E in preventing the medical conditions associated with MetS.
a-Tocopherol Deficiency and Inadequacy
Vitamin E deficiency is seldom identified in adults and is more frequently found in children, probably because they have limited stores and they are growing rapidly, so deficiency symptoms are more apparent.
It is estimated that >90% of Americans do not consume sufficient dietary vitamin E, as a-tocopherol, to meet estimated average requirements. Inadequate vitamin E status is detrimental: plasma a-tocopherol concentrations <12 mmol/L are associated with increased infection, anemia, stunting of growth, and poor outcomes during pregnancy for both the infant and the mother. When low dietary amounts of a-tocopherol are ingested, tissue a-tocopherol needs exceed amounts available, leading to increased damage to target tissues.
Adequacy of vitamin E status cannot be assessed from circulating a-tocopherol concentrations, but inadequacy can be determined from “low” values. Blood levels of a-tocopherol are very difficult to interpret because as a person ages, plasma lipid concentrations also increase and these elevations in lipids increase the plasma carriers for a-tocopherol, leading to higher circulating a-tocopherol concentrations. However, abnormal lipoprotein metabolism does not necessarily increase a-tocopherol delivery to tissues.
Furthermore, it is important to distinguish between “deficiency” and “suboptimal.” Given the activities of the different vitamin E isormers and their proposed benefits, optimal levels are quite likely to be significantly greater than simply cut-off levels for deficiency since the measurement of the consequences of suboptimal is not possible at this time. Additionally, optimal levels are likely to be dependent on many variables based on a person’s health status.
Better biomarkers of inadequate vitamin E status are needed and urinary excretion of the vitamin E metabolite a-carboxy-ethyl-hydroxychromanol may fulfill this biomarker role. Unfortunately it has not been adequately studied with regard to vitamin E status or with regard to health benefits.
The Family of Vitamin E
Vitamin E is well known for its antioxidant capacity. In actuality, Vitamin E is a family consisting of two forms, the tocopherols and the tocotrienols, each having a small difference in their molecular structure. The tocopherols were first discovered in 1922, specifically alpha-tocopherol, and are the form most familiar to people. Upon discovery in 1986, tocotrienols were first differentiated from tocopherols and found to have different properties. Current research indicates tocotrienols have greater antioxidant potential than tocopherols.
Both tocotrienols and tocopherols comes in four forms: alpha, beta, delta, and gamma but the α- forms of both tocopherols and tocotrienols are considered to be the most metabolically active. All isoforms are biologically active but α-tocopherol is the most biologically active. The most common dietary forms are α- and γ-tocopherol while only α-tocopherol is retained at high levels in plasma and tissues.
Dietary Vitamin E
The average diet contains more tocopherols than tocotrienols. γ-tocopherol is the most common in the US diet due to the higher consumption of soybeans, sesame, and corn oil, and α-tocopherol is the most common in the European diet.
Good sources of tocopherols include:
-
- Vegetable oils: olive oil, sunflower oil, rapeseed oil, corn oil, linseed oil, and soybean oil
- Nut oils
- Seeds
- Whole grains
The three most abundant sources of tocotrienols are rice bran (50%), palm oil (75%), and annatto oil (99.9%).
Good sources of tocotrienols include:
-
- rice bran oil
- oats
- barley
- hazelnuts
- rye
- wheat germ
- crude palm oil
Tocopherols
Each of the eight compounds, has shown to have some degree of antioxidant properties. However, of all the compounds, the alpha-tocopherol (aT) shows the highest physiological concentrations and only tocopherol can correct vitamin E deficiency, which suggests that tocopherol is the form of vitamin E that the body needs most to function efficiently.
Vitamin E and Metabolic Syndrome (MetS)
The benefits of vitamin E supplementation for metabolic syndrome (MetS) was evaluated in a study among MetS subjects who were non-vitamin and non-anti-oxidant users. Participants were treated with α-tocopherol (800 mg/day), γ-tocopherol (800 mg/day), combination of α-tocopherol and γ-tocopherol (800 mg each/day), or placebo for 6 weeks. The combination of α-tocopherol and γ-tocopherol was superior in reducing lipid peroxides, tumor necrosis factor- alpha (TNF-α), malondialdehyde (MDA), 4-hydroxynonenal (HNE), and high sensitivity C-reactive protein (hs-CRP) levels, suggesting their potential benefit for oxidative stress, nitrative stress, and inflammatory response in MetS.
In addition, a more recent study evaluating adults with MetS aged 20–60 years, supplementing mixed tocotrienols (400 mg/day) for 16 weeks showed beneficial effects on chronic inflammation (reduced interleukin-6 (IL-6) and TNF-α) and lipid profiles (reduced total cholesterol, LDL-C, and HDL-C).
The above studies alone are not enough to definitively conclude vitamin E impacts MetS and improves quality of life and survival. However, the current documented evidence supports the intake of naturally occurring vitamin E as a dietary supplement to prevent or treat MetS, with tocotrienols being more superior than tocopherols. Essentially, vitamin E (particularly tocotrienols) appears to be a potential medication for treating most of the clinical conditions associated with MetS.
The composition and doses of tocopherols and tocotrienols still need to be evaluated further for optimal benefits and to ensure safety.
Alpha-tocopherol (aT) as an Antioxidant
It has been shown extensively that aT acts as an antioxidant, allowing it to scavenge and remove ROS preventing oxidative damage. It protects polyunsaturated fatty acids present in cell membranes to stabilize cellular membranes them against oxidative damage. Many studies have shown a correlation between aT and reduction of inflammation and enhancement of the immune system functionality. This has stimulated interest in incorporating aT supplementation for various clinical disorders including metabolic syndrome which is associated with lower levels of aT that may indicate that the development and progression of metabolic syndrome could in part be attributed to reduced levels of aT.
Alpha-tocopherol (aT) as an Anti-inflammatory
Recent studies have shown that aT supplementation is capable of reducing inflammatory processes by reducing the expression of inflammatory agents including IL-6, TNF-a and C reactive protein while increasing antioxidant constituents. Furthermore, aT may also inhibit pathways that are activated by ROS which tend to be elevated in obese individuals and various inflammatory diseases and are reduced with aT supplementation. Thus, aT has a plausible role in reducing inflammation induced by ROS-induced oxidative damage and through its effects on immune system functionality.
Alpha-tocopherol (aT) and Arthritis
Oxidative stress is one of the underlying mechanisms contributing to cartilage degeneration in osteoarthritis as evidenced by the reduced antioxidant (including vitamin E) and increased lipid peroxidation products in the circulation and synovial fluid of the patients with osteoarthritis. The benefits of alpha-tocopherol supplementation in slowing the progression of osteoarthritis is still debatable due to the mixed outcomes in the research. High-dose vitamin E supplementation is cautioned due to its potential pro-oxidant effects. Different isoforms of vitamin E may have distinct biological effects on joint health but the studies on isoforms others than alpha-tocopherol are limited. This is a major research gap that should be addressed in future studies to validate the use of vitamin E in tackling osteoarthritis.
Alpha-tocopherol (aT) and Fatty Liver Disease
Fatty liver disease is of interest because the progression of this disorder to more serious forms of the disease is dependent on oxidative damage to lipids, suggesting that inadequate vitamin E intakes may promote disease progression.
Alpha-tocopherol (aT) to Reduce Cholesterol and Triglycerides
Research has has provided promising results for the ability of aT to reduce total cholesterol and triglycerides in metabolic syndrome and in the prevention of obesity-associated atherosclerosis. These benefits of aT are due to the reduction of low density lipoprotein (LDL), high density lipoprotein (HDL) and total cholesterol levels. In animal models of diet induced obesity, aT also diminishes the degree of fat in the liver and circulating triglycerides suggesting a likely role in the prevention of atherosclerosis and fatty liver diseases associated with obesity.
Alpha-tocopherol (aT) and Atherosclerosis
Growing evidence indicates that aT reduces oxidation of cellular membranes and reduces overall lipid content in circulation and within fat cells, suggesting aT may play a role in shifting the metabolic profile of obesity toward normalcy. Changes within fat cells in obese individuals includes an elevation of collagen deposition that may contribute to the systemic inflammation seen in obesity. Supplementation with aT may play a role in downregulating pathways associated with collagen accumulation and abnormal fat cell growth and reduce systemic inflammation.
Alpha-tocopherol (aT) and Type 2 Diabetes (T2DM)
Cumulative studies have indicated that aT supplementation reduces biomarkers of oxidative damage in T2DM patients and improves insulin sensitivity. The elevation in insulin sensitivity is attributed to the anti-inflammatory action of aT in visceral adipose tissue. Also, through scavenging of ROS, aT may may reduce the impact that persistent hyperglycemia has promoting ROS production and inflammation activation. Thus, there may be a role linking aT’s effects on inflammation that may lead to the suppression of the metabolic diseases associated with T2DM.
However, human and animal studies focused on the effectiveness of aT supplementation in aging-associated diseases, oxidative stress and inflammation have had mixed results, including beneficial and harmful effects. Recent studies suggest that vitamin C, especially in combination with vitamin E, may be helpful in reducing risk of cognitive impairment associated with Alzheimer’s .
Tocotrienols
Research also suggests independent benefits may be achieved with tocotrienols. For example, the tocotrienols are a much more bioactive form of Vitamin E, possessing 40–60 times more antioxidant activity than tocopherols. Tocotrienols also exhibit potent anti-inflammatory properties, a property that is only very weakly, if at all, observed in tocopherols.
Tocotrienols have diverse properties in addition to antioxidant and anti-inflammatory benefits, including anticancer, neuroprotective and skin protection benefits, as well as improved cognition, bone health, longevity and reduction of cholesterol levels in plasma. Tocotrienols also have been shown to improve lipid profiles, reduce total cholesterol and reduce the volume of white matter lesions in human clinical trials.
Tocotrienols and Cardiovascular Disease (CVD)
Tocotrienols improve ‘inflammaging,’ a chronic low grade inflammation in the absence of other significant medical conditions, a cardiac risk factor in the elderly. They also improve dyslipidemia and mitochondrial dysfunction, thus reducing risk for cardiovascular diseases.
Tocotrienols can lower blood levels of CRP, 20–50% more effectively than tocopherol and potentially reducing the aging risk of developing cardiovascular disease (CVD).
A new and emerging field of immunocardiology has determined that myocardial and immunological ageing processes are intimately linked and intertwined and related to activity of the immuned cells, CD4+ T cells. Tocotrienols can play a role in immunomodulation of T-cell activity and may potentially provide benefits for the heart by suppressing potentially damaging inflammatory activity by these immunologic cells.
Tocotrienols and Dyslipidemia
Dyslipidemia is defined as elevated total cholesterol levels or elevated low-density lipoprotein (LDL) cholesterol levels, or low levels of high-density lipoprotein (HDL) cholesterol. Dyslipidemia is an important risk factor for coronary heart disease (CHD) and stroke. Currently, the use of the “statins” class of drugs (Lipitor, Crestor etc.) is the gold standard for the treatment of hypercholesterolemia.
Cholesterol is produced in the body from the compound HMG-CoA via the enzyme HMG-CoA reductase. Statins mimic the structure of HMG-CoA and acts as a competitive inhibitor, thereby lowering cholesterol levels. Tocotrienols suppress the production of the enzyme HMG-CoA reductase, thereby lowering cholesterol levels.
While statins are considered the gold standard for treating hypercholesterolemia, they are associated with significant side effects. Although the incidence of these side effects is low they can nevertheless be devastating. The most common side effect is muscle pain and soreness but in more rare and extreme cases, patients may develop rhabdomyolysis, a life-threatening breakdown of muscles.
Additionally, statins have been increasingly shown to have a greater risk to the elderly by increasing the risk of developing diabetes when given high doses of statins. Thus, while one ailment is treated with reduced risk for CVD, the risk for another disease (diabetes) goes higher.
Tocotrienols can reduce triglyceride (TG) and low density lipoprotein-cholesterol (LDL-C) levels by up to 25%. Unlike statins, tocotrienols offer no risk for developing diabetes and in fact quite the opposite effect is seen. Due to its strong antioxidant activity, tocotrienols can reduce oxidative stress in the pancreas and improving insulin secretion. Additionally, tocotrienols can activate the expression of peroxisome proliferator activated receptor γ (PPARγ), a key gene crucial for improving insulin sensitivity. Hence, tocotrienols can also improve diabetes, while improving the risk for cardiovascular diseases.
Of note, when tocopherols (esp. alpha-tocopherol) are added to tocotrienols the activity of tocotrienols are reduced so they should not be taken together.
Clinical Studies with delta-Tocotrienol
Two clinical studies indicate that delta-tocotrienol, combined with antioxidant polyphenols, may curb inflammation and help manage dyslipidemia, triglycerides, or both, and help to lower high-density lipoprotein levels that contribute to the development of atherosclerosis. One placebo-controlled study was conducted in two groups of elderly people over six weeks, one with normal lipid levels, the other elevated. The study product formulation was composed of DeltaGold delta-tocotrienol from annatto along with a B-vitamin (niacin) and polyphenols.
In both groups, supplementation led to a significant drop in C-reactive protein and -glutamyl-transferase (a predictor of non-fatal myocardial infarction and fatal coronary heart disease), while increasing total antioxidant status. In the hypercholesterolemic group, the following also dropped: LDL cholesterol (by 20%-28%) and triglycerides (by 11%-18%). C-reactive protein dropped in healthy elderly subjects (by 21%-29%) and in the hypercholesterolemic elderly (by 31%-48%). No adverse effects were observed.
Of note, combining tocopherols (esp. alpha-tocopherol) with tocotrienols suppresses the benefits of tocotrienols and taking the two together should be avoided.
Statin and Tocotrienol
There has been early research on combining a statin and tocotrienol. One study reported that co-treatment with lovastatin and tocotrienols led to a synergistic effect in improving the lipid profile of hypercholesterol subjects.
Tocotrienols and Atherosclerosis
Atherosclerosis is now well recognised as a chronic in- flammatory disease. It is characterised by the accumulation of lipids and inflammatory cells in the walls of arteries, leading to the formation of atherosclerotic plaque. Vascular wall (endothelium) inflammation is the key factor in the development of atherosclerosis which results in endothelial injury and dysfunction, leading to heart attacks and strokes.
Oxidized LDL (ox-LDL) is a critical factor in the development of atherosclerotic plaque and is due to lipid peroxidation by reactive oxygen species (ROS). Dietary tocotrienols have been shown to inhibit lipid peroxidation and have a preventive role in the development of atherosclerosis. Other antioxidants, particularly CoQ-10, have been shown to protect against ox-LDLs.
Tocotrienols and High Blood Pressure (HBP)
Several recent studies have reported the benefits of dietary antioxidants in reducing blood pressure (BP), including vitamin C and tocotrienols. Studies evaluating tocotrienols in HBP are currently limited to animal studies, but the data results appear promising.
Tocotrienols and Oxidative Stress & Mitochondrial Dysfunction
Mitochondria are intracellular organelles that are the energy producing workhouses inside cells. In the production of energy, free radicals are produced including Reactive oxygen species (ROS) and reactive nitrogen species (RNS). These free radicals have been clearly established as both potentially harmful, but also potentially beneficial in different circumstances. Both ROS and RNS are normally generated by tightly regulated enzymes such as nitric oxide synthase (NOS) and nicotinamide adenine dinucleotide phosphate (NADPH) oxidase.
The chronic overproduction of ROS/RNS can be damaging to various parts of cell and tissue components and is a phenomenon linked with ageing. This is especially true when it damages important bio-molecules key to sustaining life, including lipids, proteins and DNA.
The free radical theory of ageing proposed in the 1950s and later expanded in the 1970s states that the ageing process accelerates the overproduction of these species, leading to an increased oxidative burden that needs to be cleared by the body, a condition of “oxidative stress.” The longer these ROS/RNS species persist, the higher the likelihood they cause harm by damaging tissues.
In blood vessels oxidative stress contributes to atherosclerosis and in the heart, the deterioration in mitochondrial energetics and function is a major determinant in ageing-related cardiovascular disease, including congestive heart failure. Mitochondrial dysfunction is also proposed as an element if fibromyalgia and other conditions.
See: Mitochondrial DysfunctionTocotrienols has been shown to have superior free radical scavenging activity and has been proposed as potentially helpful in suppressing oxidative stress. It should be noted that other natural compounds demonstrating powerful antioxidant and anti-inflammatory effects are likely to be beneficial for oxidative stress: curcumin, CoQ-10, resveratrol and others, (See: Antioxidants and Oxidative Stress).
Tocotrienols and Anti-Inflammation
Tocotrienols have also been evaluated for their benefit in reducing inflammation. In research studying tocotrienols’ effect on inflammatory markers with dosing of 250 mg/day of tocotrienols (identified as an optimal dose), C-reactive protein levels were reduced by 40%, and nitric oxide levels, which are aberrant in inflammatory conditions and should be reduced (only in inflammatory conditions), were also lowered by 40% in subjects.
Tocotrienols and Alzheimer’s Disease (AD)
Tocotrienols are neuroprotective and can address many aspects of Alzheimer’s Disease (AD), including oxidative stress, mitochondrial dysfunction and abnormal cholesterol synthesis. Preclinical studies show that tocotrienol reduces oxidative stress by acting as a free-radical scavenger and by promotion of mitochondrial function and cellular repair. It also prevents cellular glutamate-induced neurotoxicity which is widely implicated in the development of AD. Findings show that vitamin E protects critical fatty acids in the brain from lipid peroxidation and that improved brain vitamin E status is protective for cognitive functionred to alpha-tocopherol, tocotrienols have stronger antioxidant and anti-inflammatory activities. The structure of tocotrienols allows them to be incorporated into the cell membrane better and it has been proposed that their metabolites may be more effective than alpha-tocopherol in preventing AD. However, although tocotrienols have these better activities, alpha-tocopherol levels are higher in the brain after oral administration. At this time it is not possible to conclude which formulation is the most effective against AD.
Epidemiological studies demonstrate a significant inverse relationship between tocotrienol blood levels and the occurrence of AD: higher blood levels correlate with less likelihood of AD. At this time however, there are no clinical trials to show that tocotrienol can delay or prevent the onset of AD. While tocotrienols have the potential to be help prevent AD, additional research is necessary to validate their effiectiveness.
Tocotrienols and Weight Loss
Recent research indicates that tocotrienols may have a positive effect in reducing obesity. It has been shown that tocotrioenols inhibit production of new fat by reducing the accumulation of triglycerides (TGs) and lipid droplets.
Pharmacokinetics of Tocotrienol
Compared to tocopherols, tocotrienols are less orally bioavailable. Tocotrienols are lipid-soluble, so their absorption is enhanced with concurrent intake of dietary fat. Consumption of dietary fat ensures sufficient secretion of bile acids and lipase. Bile acids emulsify tocotrienols into microscopic droplets that provide greater surface area to be digested by lipase and absorbed into the bloodstream.
Since the oral absorption of tocotrienols depends on bile and lipase secretions which may be low and erratic, its absorption often results in poor bioavailability and effectiveness. Because of this, various methods to improve bioavailability such as using self-emulsifying drug delivery system (SEDDS) can be employed to achieve more efficient absorption.
Tocotrienols’ peak blood concentrations are reached in 3 hours after oral ingestion after which they are distributed into most organs, including serum, lung, liver, spleen, colon and adipose tissue. The transport system for vitamin E has been traditionally focused on alpha-tocopherol transport protein (α-TTP), which transports vitamin E from the liver to the blood and has specific affinity towards αTF more than other vitamin E isoforms. However, studies show that the distribution of tocotrienols can be achieved via mechanisms other than α-TTP.
Both tocopherols and tocotrienols accumulate in many tissues, including the liver, adrenal glands, and fat tissue. It is estimated that 90% of the total amount of vitamin E accumulates in the fat. Vitamin E that accumulates in fat tissue consists of about two- thirds of α-tocopherol and one-third of γ-tocopherol.
The metabolism of tocotrienols is similar to tocopherols. The elimination half-life of tocotrienols is short, approximately 2-4 hours, suggesting a twice daily supplementation dosing schedule.
α-Tocopherol Status and Requirements
The assessment of the human requirement for α-tocopherol is hampered by the rare occurrence of clinical symptoms of deficiency. Symptoms usually only develop in premature babies, infants, and adults with fat malabsorption, liver disease, or genetic diseases.
Recommended Daily Allowance of Vitamin E
Currntly, daily allowance recommendations are 8–15 mg of α-tocopherol or an α-tocopherol equivalent for women and men. There are significant differences in α-tocopherol intake in different countries, varying from 8 to 10 mg/person/day in Finland, Iceland, Japan, and New Zealand, to 20 to 25 mg/person/day in France, Greece and Spain. However, in a study that focused on a comparison of vitamin E intake in different subpopulations, over 80% were below the RDA of 15 mg/day.
The optimum dose and blood levels of tocotrienols required for neuroprotection and other benefits are unknown at this moment.
Blood Levels
The concentration of α-tocopherol in blood (serum) is the main method used to assess α-tocopherol status. Levels of α-tocopherol in serum below 9 μmol/L in men and below 12 μmol/L in women are considered as deficiency. There is strong evidence that men with higher serum α-tocopherol levels (≥14.2 mg/L vs. <9.3 mg/L) have lower overall mortality and lower mortality from cardiovascular disease (CVD), heart disease, stroke, cancer, and respiratory disease. No reference values have been established for the other forms of vitamin E. Additionally, there is a lack of correlation between dietary vitamin E intakes and blood levels of a-tocopherol.
In cancer prevention studies, higher blood levels of α-tocopherol were associated with lower mortality. The lowest total mortality was observed at the concentration of α-tocopherol at the level of 30 μmol/L in the blood serum. Additionally, other results of observational studies showed that at the point of 30 μmol/L and above, the concentration of α-tocopherol in the serum has a positive effect on human health. However, only 21% of reported populations reach this threshold, possibly indicating a generally low vitamin E (α-tocopherol) nutritional status worldwide.
According to some, plasma α-tocopherol concentration is not a reliable marker for the assessment of vitamin E status, especially in subjects with an abnormal lipids profile. Therefore, adjusting α-tocopherol to plasma lipids and lipoproteins is recommended. However, it is not widely used, and it may be a cause of overinterpretation and ambiguity of the described results about α-tocopherol status.
Plasma α-tocopherol concentration may be modified by several factors such as age, gender, lifestyle, low circulating lipid levels, genetic variation and variation in the absorption, metabolism, and excretion of vitamin E, as well as by obesity, metabolic syndrome, or high levels of oxidative stress. Additionally, the assessment of vitamin E status is also difficult because it is a fat-soluble vitamin, which is stored in adipose tissue.
In patients with excess body weight who performed a weight reduction diet for six weeks, asignificant reduction in blood plasma α-tocopherol was found and almost 80% of them levels below 20 μmol/L. This may indicate an increased risk for cardiovascular diseases and low antioxidant protection. It is unclear whether the above-mentioned differences in distribution of the α-tocopherol and other isoforms are due to insufficient intake, changes in metabolism in obese individuals, or due to these two factors.
Obesity and Vitamin E Status
There is a significant relationship between the content of adipose tissue (fat) in the body and the demand and metabolism of tocopherols and tocotrienols, mainly α-tocopherol. An excessive level of adipose tissue creates chronic inflammation with increased production of cytokines, proteins, and immune response mediators, leading to the activation of inflammatory pathways.
Chronic, low-grade inflammation in obesity leads to increased oxidative stress and a disrupted balance of oxidants and antioxidants in the body. Bioactive dietary antioxidants such as tocopherols and tocotrienols can prevent damage caused by inflammation and reactive oxygen species, thereby reducing the negative effects of obesity. On the other hand, an excess of adipose tissue may generate in the body an increased demand for antioxidants, which may cause their greater utilization, leading to their decreased concentration in the blood.
More research is needed concerning the relationships between obesity and blood α-tocopherol, other vitamin E isomers, or their metabolites concentration. Significantly higher blood levels of γ-tocopherol in people with obesity compared with normal body weight and a lack of differences between these two groups for α-tocopherol. It has also been shown that in people with metabolic syndrome and obesity, the level of excreted α-tocopherol metabolites as well as the plasma level of α-tocopherol were lower, and the level of oxidative stress increased.
Tocotrienols and Safety
Palm tocotrienol-rich fraction (TRF) has been labelled “GRAS” (Generally Regarded as Safe) status and determined safe for human consumption by the FDA in 2010. Clinical trials with doses of approximately 50–400 mg/day for durations of 2 weeks to 18 months reported no adverse effects, even in the elderly.
Tocotrienols and Bioavailability
The amount of vitamin E absorbed depends on the food matrix that supplies it. Alcohol and dietary fiber inhibit the absorption of vitamin E. Studies have shown low bioavailability of vitamin E isoforms from the apples matrix and high bioavailability from bananas and bread matrix.
Tocotrienols’ bioavailability can be enhanced with emulsions that increase absorption. Another solution for increasing tocotrienols’ bioavailability is to take tocotrienols with a meal.
Tocotrienols and the Future
Research has established that not all vitamin E isomers are the same and that tocotrienols have distinctive functions from tocopherols. There is a growing body of evidence supporting tocotrienols’ benefits for chronic conditions. Given that research indicates that tocopherol does not enhance the function of tocotrienol, but rather antagonizes it, manufacturers should formulate with delta- and gamma- tocotrienols, without tocopherol or with the lowest amount of alpha-tocopherol possible. It remains early to establish specific recommendations for supplementing with tocotrienols and at what dose.
Comparison between the Effects of Tocotrienol and Tocopherol
The different isomers of Vitamin E possess protective effects on a variety of diseases in varying degrees. Tocotrienols and tocopherols offer many benefits, mainly by alleviating inflammatory response, oxidative stress, mitochondrial dysfunction, and abnormal cholesterol synthesis. The anti-hyperlipidemic, anti-osteoporotic, anti-hyperglycemic, anti-inflammatory, anti-oxidative, neuroprotective, gastroprotective, and cardioprotective effects of Tocotrienols seem to be more superior or as effective compared to tocopherols.
HMGCR inhibition
HMGCR inhibition is one of the biological actions of tocotrienols which is not observed with tocopherols.
Antioxidant Activity
Antioxidant activity is one of the main molecular actions of the isomers of Vitamin E. Both tocotrienols and tocopherols scavenge free radicals but tocotrienols have better membrane antioxidant activity compared to tocopherols. δ-tocotrienol exerts greater inhibition on lipid peroxidation and ROS production than γ-tocotrienol and α-tocotrienol.
Reducing Cholesterol
The hypocholesterolemic actions between tocotrienols and tocopherols have been compared in several studies. There isvidence that γ-tocotrienol had higher efficacy than mixed tocotrienol (containing 9.9% γ-tocopherol) in lowering TC and LDL-C. It was also found that α-tocopherol is less effective in improving lipid metabolism as compared to TRF (containing a mixture of tocotrienols and tocopherols), γ-tocotrienol, and δ-tocotrienol. In humans, tocotrienols but not α-tocopherol, reduced TC and LDL-C in subjects with mild hypercholesterolemia.
Cardioprotective Effects
In cardiovascular diseases, γ-tocotrienol exhibits the best cardioprotective effect in myocardial ischemia-reperfusion injury model, followed by α-tocotrienol and δ-tocotrienol. In animal studiess, δ-tocotrienol was capable of improving more metabolic abnormalities than γ-tocotrienol, while α-tocotrienol and α-tocopherol were the least effective.
Bone Health
With regards to bone health, previous studies showed that two different forms of vitamin E (palm tocotrienols mixture with α-tocopherol) were comparable in preserving bone microarchitecture and bone density in postmenopausal osteoporosis rat model. Otherwise, some studies reported better outcomes on the skeleton when normal or osteoporotic animals were supplemented with tocotrienols compared to α-tocopherol. In fractured rat model, tocotrienols was also better than α-tocopherol in improving bone strength of the fractured callous in estrogen-deficient rats. Mechanistically, tocotrienols was shown to have more potent anti-inflammatory and anti-oxidative properties, which were responsible for its superior skeletal-promoting effects in animals.
Metabolic Regulation
In terms of metabolic regulation, tocotrienols are more effective than α-tocopherol at similar doses in normalizing body weight and glucose level in diabetic rats. These observations might be attributed to the higher efficacy of tocotrienols in reducing inflammatory response and lipid peroxidation. α-, γ-, and δ-tocotrienol improve insulin synthesis, wherein δ-tocotrienol is the most potent isoform, followed by γ- and α-tocotrienol. Additionally, the anti-adipogenic activities of each tocotrienols were reported by following the rank order of γ > δ > β > α-tocotrienol.
Cancer
In anticancer studies, tocotrienols have significantly greater anticancer activities than their corresponding tocopherol isoforms.
Taken together, vitamin E isomers display beneficial properties in preventing various conditions in general even though the different effects between the isomers of tocotrienols and tocotrienol are present.
Safety of Tocotrienol and Tocopherol
Vitamin E is relatively safe, as has been reported in animal studies, vitamin E is not mutagenic, carcinogenic, or teratogenic. At extremely high doses, way above the usual supplemental dose, bleeding and clotting time in mice were increased, thus the concurrent use of vitamin E isomers with other anticoagulants should be used with caution. Vitamin E supplementation has been associated with hemorrhagic stroke in a meta-analysis. In another human study, oral consumption of high dose of vitamin E (3200 IU/day) is well-tolerated by adults. Since vitamin E, α-tocopherol, and mixed tocotrienols are widely available as over-the-counter supplements, it is hard to regulate their use. but it is important that appropriate doses of vitamin E be employed.
Carotenoids
Carotenoids are molecules found in red, yellow, and orange fruits and vegetables and are subdivided into carotenes and xanthophylls. Carotenes differ in structure from xanthophylls by the absence of oxygen groups, while xanthophylls may have multiple oxygen groups and are more soluble in water. The complexity of their actions on lipid membranes is due to the unique action of each carotenoid to various compositions of membranes. In the small intestine, carotenoids are emulsified into micelles and their absorptions are facilitated by cell surface proteins. After absorption, carotenoids are transported to the liver and then released into blood. Carotenes are usually packaged into LDL, while xanthophylls are typically packaged into HDL. Both types of carotenoids are known for their functions in vision, but growing evidence suggests they have anti-obesity activities).
Beta-carotene, Precursor to Vitamin A
Beta-carotene is a yellow-red-orange pigment found in plants and fruits, especially carrots and colorful vegetables that is a vitamin A precursor. In a human study with 29,000 participants, elevated serum beta-carotene levels were associated with lower cardiovascular and heart disease. Other human studies have demonstrated that higher beta-carotene is associated with lower incidence of metabolic syndrome and lower body weight.
- Beta-carotene is converted into vitamin A, an essential vitamin
- Vitamin A is toxic at high levels
- Beta-carotene is an antioxidant
- Foods rich in vitamin A include onions, carrots, peas, spinach and squash
- Some evidence suggests that beta-carotene might slow cognitive decline
- Beta-carotene supplements interact with certain drugs, including statins
- Beta-carotene might help older people retain their lung strength as they age.
Antioxidants – Summary
Widespread oxidative free radicals are major contributors to the manifestations of oxidative stress, particularly in the presence of obesity. This argues for the potential need for antioxidant supplementation in the obese population as antioxidants have been shown to reduce the manifestations of oxidative stress. Given the ease of availability of these antioxidants as dietary constituents and over-the-counter supplements, individuals are able to reap their benefits at minimal cost for optimal potential therapy.
Antioxidants are able to scavenge ROS, thus reducing oxidative stress but each individual antioxidant has its own characteristic mechanisms to provide benefit and each antioxidant is distinct in terms of the pathways they inhibit or stimulate. For instance, the lipophilicity of the E-vitamins and carotenoids enable them to offer more beneficial effects within lipid membranes and lipoproteins compared to others. Some of these antioxidants are able to act through indirect and direct mechanisms allowing for the potential to act across various pathways such as NAC, various polyphenols and carotenoids. The specific properties of each individual antioxidant provides clues to which metabolic dysfunction they are optimally able to respond to.
References
Systemic Inflammation – Overviews
-
- Systemic Inflammatory Response Syndrome – PubMed – 2022
- Chronic inflammation in the etiology of disease across the life span – 2019
- Pathophysiology and Therapeutic Perspectives of Oxidative Stress and Neurodegenerative Diseases – A Narrative Review – 2020.pdf
- A Pharmacological Rationale to Reduce the Incidence of Opioid Induced Tolerance and Hyperalgesia – A Review – 2018
- Pathophysiology of musculoskeletal pain – a narrative review – 2021 Cannabinoids in Chronic Pain: Therapeutic Potential Through Microglia Modulation – 2022
- Targeting inflammation as a treatment modality for neuropathic pain in spinal cord injury: a randomized clinical trial – 2016
- How Systemic Inflammation Affects Your Brain & Central Nervous System Cannabinoid Receptors and Their Relationship With Chronic Pain: A Narrative Review – 2020
- The Neuroimmunology of Chronic Pain: From Rodents to Humans – 2021
- Cannabis Has Low Risks, Small Benefits for All Types of Chronic Pain, Say New International Guidelines – 2022
- Microglia activation states and cannabinoid system Therapeutic implications – PubMed -2016
- Inflamm-aging does not simply reflect increases in pro-inflammatory markers – 2014
- Systemic Inflammation Predicts All-Cause Mortality: A Glasgow Inflammation Outcome Study – 2015
Systemic Inflammation – Diet
-
- The Effects of Sesame Consumption on Glycemic Control in Adults: A Systematic Review and Meta-Analysis of Randomized Clinical Trial – 2021
- Effects of Sesame Consumption on Inflammatory Biomarkers in Humans: A Systematic Review and Meta-Analysis of Randomized Controlled Trials – 2021
- Sesame oil and vitamin E co-administration may improve cardiometabolic risk factors in patients with metabolic syndrome a randomized clinical trial – PubMed – 2019
- Vitamin C: A Review on its Role in the Management of Metabolic Syndrome – 2020
- Obesity–An Update on the Basic Pathophysiology and Review of Recent Therapeutic Advances – 2021
- Diet-Derived Antioxidants and Their Role in Inflammation, Obesity and Gut Microbiota Modulation – 2021
- The Role of Vitamins in Non-Alcoholic Fatty Liver Disease: A Systematic Review – 2921
- Spices, Condiments, Extra Virgin Olive Oil and Aromas as Not Only Flavorings, but Precious Allies for Our Wellbeing – 2021
- Anti-inflammatory diet and inflammatory bowel disease: what clinicians and patients should know? – 2021
- Current nutrition reports Author Manuscript HHS Public Access
- The Role of Vitamin K in Chronic Aging Diseases: Inflammation, Cardiovascular Disease, and Osteoarthritis – 2016
- Role of PPARγ in the nutritional and pharmacological actions of carotenoids – 2018
- Role of mitochondria, oxidative stress and the response to antioxidants in myalgic encephalomyelitis:chronic fatigue syndrome: A possible approach to SARS-CoV-2 ‘long-haulers’? – 2021
- β-Carotene Status Is Associated with Inflammation and Two Components of Metabolic Syndrome in Patients with and without Osteoarthritis – 2021
- Anti-inflammatory Activity of β-Carotene, Lycopene and Tri-n-butylborane, a Scavenger of Reactive Oxygen Species – 2018
- Role of Vitamin A in the Immune System – 2018
- ALA Nutritional Benefits – 2008
Vitamin E – Tocotrienol
-
- Tocotrienol is a cardioprotective agent against ageing-associated cardiovascular disease and its associated morbidities. – 2016.pdf
- Vitamin E Oxidized LDL.pdf
- Tocotrienols – Vitamin E Beyond Tocopherols – 2006.pdf
- Tocotrienols, the Vitamin E of the 21st Century: It’s Potential Against Cancer and Other Chronic Diseases – 2010
- Metabolism of natural forms of vitamin E and biological actions of vitamin E metabolites – 2022
- Tocopherols and Tocotrienols—Bioactive Dietary Compounds; What Is Certain, What Is Doubt? – 2021
- Enjoy Carefully: The Multifaceted Role of Vitamin E in Neuro-Nutrition – 2021
- Tocopherols, tocotrienols and tocomonoenols: Many similar molecules but only one vitamin E – 2019.pdf
- An Interactive Review on the Role of Tocotrienols in the Neurodegenerative Disorders – 2021
- Potential Role of Tocotrienols on Non-Communicable Diseases: A Review of Current Evidence – 2020
- The Implication of Reactive Oxygen Species and Antioxidants in Knee Osteoarthritis – 2021
- Tocotrienols the unsaturated sidekick shifting new paradigms in vitamin E therapeutics – PubMed – 2017
- Hidden Hunger of Vitamin E among Healthy College Students: A Cross- Sectional Study – 2021
- The Effects of Sesame Consumption on Glycemic Control in Adults: A Systematic Review and Meta-Analysis of Randomized Clinical Trial – 2021
- Effects of Sesame Consumption on Inflammatory Biomarkers in Humans: A Systematic Review and Meta-Analysis of Randomized Controlled Trials – 2021
- Sesame oil and vitamin E co-administration may improve cardiometabolic risk factors in patients with metabolic syndrome a randomized clinical trial – PubMed – 2019
- Effects of tocotrienols supplementation on markers of inflammation and oxidative stress: A systematic review and meta-analysis of randomized controlled trials – 2021
- Metabolic Syndrome, Cognitive Impairment and the Role of Diet: A Narrative Review – 2022
- Mechanistic Effects of Vitamin D Supplementation on Metabolic Syndrome Components in Patients with or without Vitamin D Deficiency – 2020
- Vitamin E As a Potential Interventional Treatment for Metabolic Syndrome: Evidence from Animal and Human Studies – 2021
- A Review on the Relationship between Tocotrienol and Alzheimer Disease – 2018.pdf
- The Role of Vitamin E in Preventing and Treating Osteoarthritis – A Review of the Current Evidence – 2018
- The Role of Tocotrienol in Preventing Male Osteoporosis-A Review of Current Evidence – 2019
- Association between serum Vitamin E concentrations and the presence of Metabolic Syndrome: A population-based cohort study – 2021
- The Role of Tocotrienol in Protecting Against Metabolic Diseases – 2019
- Therapeutic Efficacy of Antioxidants in Ameliorating Obesity Phenotype and Associated Comorbidities – 2020
- Pharmacology and Pharmacokinetics of Vitamin E: Nanoformulations to Enhance Bioavailability – 2020
- Vitamin E inadequacy in humans: causes and consequences – 2014
Oxidative Stress – Overviews
-
- oxidative-stress-implications-in-the-affective-disorders-main-biomarkers-animal-models-relevance-genetic-perspectives-and-antioxidant-approaches-2016
- oxidative-stress-in-health-and-disease-the-therapeutic-potential-of-nrf2-activation-2011
- adaptive-cellular-stress-pathways-as-therapeutic-targets-of-dietary-phytochemicals-focus-on-the-nervous-system-2014
- oxidative-stress-a-cause-and-therapeutic-target-of-diabetic-complications-2010
- a-randomized-trial-of-glutamine-and-antioxidants-in-critically-ill-patients-2013
- Inflammation, Oxidative Stress, and Antioxidants Contribute to Selected Sleep Quality and Cardiometabolic Health Relationships – 2015
- Mitohormesis: Promoting Health and Lifespan by Increased Levels of Reactive Oxygen Species (ROS) – 2014
- Oxidative Stress – Harms and Benefits for Human Health – 2017
Oxidative Stress – Aging
Oxidative Stress – Anxiety and Depression
-
- Markers of Oxidative Stress and Neuroprogression in Depression Disorder – 2015
- Neuroinflammation and Depression – Microglia Activation, Extracellular Microvesicles and microRNA Dysregulation – 2015
- Novel Therapeutic Targets in Depression and Anxiety – Antioxidants as a Candidate Treatment
- Oxidative:nitrosative stress and antidepressants: targets for novel antidepressants. – PubMed – NCBI
Oxidative Stress – Cardiovascular
Oxidative Stress – Diabetic Neuropathy
Oxidative Stress – Fibromyalgia
-
- Oxidative Stress Correlates with Headache Symptoms in Fibromyalgia – Coenzyme Q10 Effect on Clinical Improvement 2012
- Free radicals and antioxidants in primary fibromyalgia: an oxidative stress disorder? – PubMed – NCBI
- Current concepts in the pathophysiology of fibromyalgia: the potential role of oxidative stress and nitric oxide. – PubMed – NCBI
- Oxidative Stress in Fibromyalgia – Pathophysiology and Clinical Implications – 2011
- Oxidative Stress in Fibromyalgia and its Relationship to Symptoms – 2009
- Clinical Symptoms in Fibromyalgia Are Better Associated to Lipid Peroxidation Levels in Blood Mononuclear Cells Rather than in Plasma
- Evidence of central inflammation in fibromyalgia — Increased cerebrospinal fluid interleukin-8 levels 2012
- Oxidative Stress in Fibromyalgia – Pathophysiology and Clinical Implications – 2011
- Vitamins C and E treatment combined with exercise modulates oxidative stress markers in blood of patients with fibromyalgia: a controlled clinical … – PubMed – NCBI
- Total antioxidant capacity and the severity of the pain in patients with fibromyalgia. – PubMed – NCBI
- Stress, the stress response system, and fibromyalgia
- Serum prolidase enzyme activity and oxidative status in patients with fibromyalgia. – PubMed – NCBI
- Serum ischemia-modified albumin and malondialdehyde levels and superoxide dismutase activity in patients with fibromyalgia. – 2014 – PubMed – NCBI
- Pathophysiology and antioxidant status of patients with fibromyalgia. 2011 – PubMed – NCBI
- Metformin and caloric restriction induce an AMPK-dependent restoration of mitochondrial dysfunction in fibroblasts from Fibromyalgia patients. 2015 – PubMed – NCBI
- Fibromyalgia and chronic fatigue: the underlying biology and related theoretical issues. – PubMed – NCBI
- Antioxidant status, lipid peroxidation and nitric oxide in fibromyalgia: etiologic and therapeutic concerns. 2006 – PubMed – NCBI
Oxidative Stress – Fibromyalgia & Mitochondria
-
- Serum antioxidants and nitric oxide levels in fibromyalgia: a controlled study. 2009 – PubMed – NCBI
- Mitochondrial dysfunction and mitophagy activation in blood mononuclear cells of fibromyalgia patients – implications in the pathogenesis of the disease
- Could mitochondrial dysfunction be a differentiating marker between chronic fatigue syndrome and fibromyalgia? – PubMed – NCBI
- Is Inflammation a Mitochondrial Dysfunction-Dependent Event in Fibromyalgia? – 2012
- The role of mitochondrial dysfunctions due to oxidative and nitrosative stress in the chronic pain or chronic fatigue syndromes and fibromyalgia – 2013 – PubMed – NCBI
- Mitohormesis: Promoting Health and Lifespan by Increased Levels of Reactive Oxygen Species (ROS) – 2014
- Oxidative stress and mitochondrial dysfunction in fibromyalgia. – PubMed – NCBIOxidative-Stress-in-Fibromyalgia-and-its-Relationship-to-Symptoms-2009
- The-role-of-mitochondrial-dysfunctions-due-to-oxidative-and-nitrosative-stress-in-the-chronic-pain-or-chronic-fatigue-syndromes-and-fibromyalgia-patients-20131
Oxidative Stress – Mitochondria
-
- Mitohormesis: Promoting Health and Lifespan by Increased Levels of Reactive Oxygen Species (ROS) – 2014
- The Mitochondrial Basis of Aging and Age-Related Disorders – 2017
- Mitochondrion-Permeable Antioxidants to Treat ROS-Burst-Mediated Acute Diseases – 2016 no highlights
- Current Experience in Testing Mitochondrial Nutrients in Disorders Featuring Oxidative Stress and Mitochondrial Dysfunction
- Mitochondrial biogenesis: pharmacological approaches. – PubMed – NCBI
- The mitochondrial cocktail: rationale for combined nutraceutical therapy in mitochondrial cytopathies. – PubMed – NCBI
- Oxidative Stress and Mitochondrial Dysfunction across Broad-Ranging Pathologies – Toward Mitochondria-Targeted Clinical Strategies
- Daily Nutritional Dose Supplementation with Antioxidant Nutrients and Phytochemicals Improves DNA and LDL Stability
Oxidative Stress – Neurodegenerative Diseases
Oxidative Stress – Pain
-
- Roles of Reactive Oxygen and Nitrogen Species in Pain – 2011
- Clinical Relevance of Biomarkers of Oxidative Stress – 2015
- The Interplay between Oxidative Stress, Exercise, and Pain in Health and Disease – Potential Role of Autonomic Regulation and Epigenetic Mechanisms – 2020
- Neuropathic Pain – Delving into the Oxidative Origin and the Possible Implication of Transient Receptor Potential Channels – 2018
- Neuroprotective Effect of Antioxidants in the Brain – 2020
- Dietary Patterns and Interventions to Alleviate Chronic Pain – 2020
Oxidative Stress – Peripheral Neuropathy
Emphasis on Education
Accurate Clinic promotes patient education as the foundation of it’s medical care. In Dr. Ehlenberger’s integrative approach to patient care, including conventional and complementary and alternative medical (CAM) treatments, he may encourage or provide advice about the use of supplements. However, the specifics of choice of supplement, dosing and duration of treatment should be individualized through discussion with Dr. Ehlenberger. The following information and reference articles are presented to provide the reader with some of the latest research to facilitate evidence-based, informed decisions regarding the use of conventional as well as CAM treatments.
For medical-legal reasons, access to these links is limited to patients enrolled in an Accurate Clinic medical program.
Should you wish more information regarding any of the subjects listed – or not listed – here, please contact Dr. Ehlenberger. He has literally thousands of published articles to share on hundreds of topics associated with pain management, weight loss, nutrition, addiction recovery and emergency medicine. It would take years for you to read them, as it did him.
For more information, please contact Accurate Clinic.
Supplements recommended by Dr. Ehlenberger may be purchased commercially online or at Accurate Clinic.
Please read about our statement regarding the sale of products recommended by Dr. Ehlenberger.
Accurate Supplement Prices
.